Optical devices using shaped optical fibers and methods for making optical devices with shaped optical fibers
[0001] This application is related to co-pending U.S. patent application Ser. Nos. 09/515,187 and 09/515,448, and claims priority to U.S. Provisional Application No. 60/315,960, filed Aug. 29, 2001. [0002] This invention relates to methods for making optical fiber devices using shaped optical fibers or optical fibers with multiple core regions. The fibers have shapes and/or surface features with a known orientation to a transverse axis of the fiber. [0003] To maintain or preserve the polarization properties of a signal in an optical fiber, the optical properties of the fiber may be made anisotropic. In highly birefringent single core optical fibers the waveguiding region formed by the cladding and core regions in the fiber define two transverse orthogonal axes, which permit the de-coupling of waves polarized along those axes. If a signal launched into these fibers has its polarization aligned with one of these transverse axes, the polarization tends to remain aligned with that axis as the signals are propagated though the fiber. This preserves the polarization of the signal. Highly birefringent optical fibers such as, for example, polarization maintaining (PM) and polarizing (PZ) fibers, require precise alignment of their transverse orthogonal axes when they are joined to other similar fibers, interfaced with other polarized sources or detectors, have a Bragg grating written into their core regions, or are treated during other manufacturing processes. Identification and alignment of the fiber's transverse axes requires a considerable amount of time and complex equipment. In addition, errors in locating the transverse axes cause poor performance in optical fiber devices using birefringent fibers. [0004] In addition, whether birefringent or not, optical fibers may include features that are not rotationally symmetric. For example, to improve the coupling efficiency of a single mode or a PM/PZ optical fiber to an optoelectronic semiconductor device, the fiber may include a lensed tip. As a specific example, to achieve maximum coupling efficiency between an optical fiber and erbium-doped optical fiber preamplifier pump laser, the tip of the fiber may be fabricated with a wedge-shaped lens. Since such semiconductor devices emit an optical beam that is highly elliptical, there is inherent rotational angle dependence when coupling the output beam of the device into the wedge-shaped lens on the fiber tip. Therefore, to achieve maximum coupling efficiency, some means of rotational alignment is required between the relative angles of the emission ellipse and the wedge-face direction of the lens. Errors in this alignment procedure introduce variability into device coupling, and efforts to reduce and/or eliminate these alignment errors increase production costs. [0005] U.S. patent application Ser. Nos. 09/515,187 and 09/515,448 describe birefringent optical fibers with an outer periphery that may be shaped to provide an alignment feature or an external rotational reference. The non-circular cross-sectional shape of the fiber, or features on the outer surface of the fiber, provide an easily visible, “passive” means of locating an internal region in the fiber, such as the fiber's transverse, orthogonal birefringent axes, or an external surface feature. This allows the fibers to be easily rotationally aligned with respect to a device while maintaining their internal regions or surface features in a known orientation with respect to the device. [0006] The present invention is directed to optical devices using shaped fibers and methods for using them to make optical devices. [0007] In one aspect, the invention is a method for sensitizing an optical fiber, including: (a) providing a section of a shaped optical fiber having a waveguiding region with a known internal geometry, wherein the waveguiding region is oriented at a known angle with respect to one of: (i) a portion of an outer surface of the fiber, and (ii) one or more features on the outer surface of the fiber; and (b) passing the fiber between a first electrode and a second electrode such that an electric field is applied to the waveguiding regions wherein at least one of the first and second electrodes have a feature that engages at least one of the portion of the outer surface of the fiber and the feature of the fiber to maintain the orientation of the waveguiding region of the optical fiber with respect to the applied electric field. [0008] In a second aspect, the invention is a method for making an electric field sensor, including: (a) providing a shaped optical fiber having a waveguiding region with transverse axes, wherein the axes are oriented at a known angle with respect to one of: (i) a portion of an outer surface of the fiber, and (ii) one or more features on the outer surface of the fiber; (b) poling the fiber; and (c) wrapping the fiber about a cylindrical insulator, wherein an outer surface of the insulator includes a feature that engages at least one of the portion of the outer surface of the fiber and the feature of the fiber to maintain the orientation of the waveguiding region of the optical fiber with respect to an applied electric field, and (d), applying an electric field to the optical fiber to produce electro-optic axes exhibiting a phase difference in light transmitted through the optical fiber, wherein the phase difference has a known orientation with respect to the transverse axes of the fiber. [0009] In a third aspect, the invention is a method for making an optical fiber Bragg grating, including providing an orientation device having a surface feature; inserting into the surface feature of the orientation device a shaped optical fiber having a waveguiding region with transverse axes, wherein the axes are oriented at a known angle with respect to one of: (i) a portion of an outer surface of the fiber, and (ii) one or more features on the outer surface of the fiber; and writing a Bragg grating in the waveguiding region at a known angle with respect to the transverse axes of the waveguiding region. [0010] In a fourth aspect, the invention is a method for altering the birefringence of an optical fiber, including: (a) providing a shaped optical fiber having a waveguiding region with transverse axes, wherein the axes are oriented at a known angle with respect to one of: (i) a portion of an outer surface of the fiber, and (ii) one or more features on the outer surface of the fiber, (b) straining the optical fiber in a device having a surface with a feature that engages at least one of the portion of the outer surface of the fiber and the feature of the fiber to maintain the orientation of the waveguiding region of the fiber with respect to the device. [0011] In a fifth aspect, the invention is a method for making a polarization splitter or combiner, including: (a) providing a first shaped birefringent optical fiber with a first surface feature having a known orientation with respect to the principal axes of the first fiber; (b) providing a second shaped birefringent optical fiber with a second surface feature having a known orientation with respect to the principal axes of the second fiber; and (c) fusing the first fiber and the second fiber together in an arrangement such that the principal axes of the first fiber are aligned at a known angle with respect to the principal axes of the second fiber. [0012] In a sixth aspect, the invention is a method for making a polarization maintaining coupler, including: (a) providing a first alignment fixture with a first alignment feature; (b) providing a second alignment fixture with a second alignment feature; (c) mounting in the first alignment feature a first surface feature of a first shaped birefringent optical fiber, wherein the first surface feature has a known orientation with respect to the principal axes of the first fiber; (d) mounting in the second alignment feature a second surface feature of a second shaped birefringent optical fiber, wherein the second surface feature has a known orientation with respect to the principal axes of the second fiber; and (e) fusing the first fiber and the second fiber together such that the principal axes of the first fiber are aligned at a known angle with respect to the principal axes of the second fiber. [0013] In a seventh aspect, the invention is a twin-core optical fiber having a first core and a second core, wherein the fiber has at least one of a cross-sectional shape and a surface feature oriented at a known angle with respect to a line between the first core and the second core. [0014] In an eighth aspect, the invention is a birefringent optical fiber including a Bragg grating, wherein the fiber has a non-circular cross-sectional shape. [0015] In a ninth aspect, the invention is an optical fiber including an endface with a nonspherical lens having a lens axis transverse to the longitudinal axis of the fiber, wherein the lens axis has a known orientation with respect to at least one of: (i) an outer surface, and (ii) a surface feature of, the fiber. [0016] In a tenth aspect, the invention is an optical interconnection between two optical fibers with dissimilar cross-sectional shapes, including: [0017] (a) a first fiber having a first optical core, a first effective diameter, and a first endface with an essentially round outer surface; [0018] (b) a second fiber having a second optical core, a second effective diameter, and a second endface with a non-round outer surface; and [0019] (c) an alignment fixture having at least one continuous surface, wherein the first effective diameter and the second effective diameter are not substantially equal, and when the first and second fibers are placed in the alignment fixture with their respective endfaces in abutting relationship in contact with the continuous surface, the first and second optical cores are in optical communication. [0020] The details of one or more embodiments of the invention are set forth in the accompanying drawings and the description below. Other features, objects, and advantages of the invention will be apparent from the description and drawings, and from the claims. [0021] [0022] [0023] [0024] [0025] [0026] [0027] [0028] [0029] [0030] [0031] [0032] [0033] [0034] [0035] [0036] [0037] [0038] [0039] [0040] [0041] [0042] Like reference symbols in the various drawings indicate like elements. [0043] Copending U.S. patent application Ser. Nos. 09/515,187 and 09/515,448, incorporated herein by reference, describe specially shaped highly birefringent optical fibers. These fibers have an outer periphery that may be shaped independently of the cross sectional geometry of the highly birefringent waveguiding region. The cross-sectional shape of these optical fibers may be a specific non-circular shape, such as a diamond, a triangle, or the like, or may include an outer surface with one or more features such as, for example, flat sides, bumps, or slots. The transverse axes of the waveguiding region of the fiber preferably have a known orientation with respect to a portion of the outer surface of the fiber or with respect to one or more features on the outer surface of the fiber. The cross-sectional shape of the fiber or features on the fiber surface provide an easily visible, “passive” means of locating the fiber's transverse, orthogonal birefringent axes, which allows the fibers to be easily aligned with other devices without time consuming alignment steps and expensive equipment. [0044] The preform used to make the shaped fibers described in the '187 and '448 applications may be made by a modified chemical vapor deposition (MCVD) process and may include at least one core region having surrounding cladding regions with substantially circular cross sections. However, any preform with a substantially round cross section that is designed to produce an optical fiber with highly birefringent, single mode operation, may be used. Examples include preforms with diametrically opposed stress-applying regions in the cladding, preforms with a core and/or a cladding having a substantially elliptical cross section, and preforms with multiple core regions. Any known process may be used to make these preforms. For example, a rod in tube or outside vapor deposition (OVD) process may be used to form a preform to make an optical fiber with a trade designation PANDA having a substantially circular cross section, a MCVD, an OVD process may be used to form a bow tie preform with a substantially circular cross section, or MCVD may be used to fabricate a preform with a substantially circular cross sectional shape having a core with a substantially elliptical cross sectional shape. [0045] These shaped birefringent fibers are useful in any application where the location of the transverse birefringent axes of the core in a birefringent fiber, or the location of the electro-optic axes in a poled fiber, must be determined with accuracy. Shaped fibers are also useful where the core locations in a dual core or multi-core single mode fiber must be accurately determined. [0046] For example, poled single mode or PM fibers may be used as a transducer in an electro-optic electric field sensor. Prior to use in the sensor, an external electric field is applied along the entire length of a short section of the fiber in a plane normal to the direction of light propagation, which sensitizes the fiber by maximizing its electo-optic coefficient and second order nonlinearity along the direction of the poling field. The sensitized fiber is then helically or spirally coiled on a dielectric cylinder capped by flat electrodes arranged at the two ends of the cylinder. The principal electro-optic axes of the coiled fiber are oriented in a constant direction with respect to an electrical field applied to the electrodes. When the electric field is applied to the electrodes, an electro-optic effect induces a phase difference in the orthogonal components of a light wave traveling down the fiber. In a voltage sensor, for example, this phase difference is directly proportional to the potential difference applied between the electrodes. [0047] These sensors have not been commercially successful in part because there is neither a fast nor an economical way to maintain the alignment of the principal electro-optic axes of a poled single mode or PM fiber as it is sensitized along its entire length. In addition, it is difficult to accurately coil the sensitized fiber along the dielectric cylinder while maintaining the principal axes in a desired direction with respect to the electrical field to be measured. It has been recognized that an elliptically cored PM fiber with a longitudinal flat having a predetermined orientation with respect to the principal axes of the fiber may provide an accurate alignment of the principal axes with respect to the applied field as the fiber is wrapped about the cylinder. Unfortunately, if the flat is placed against the cylinder, the principal electro-optic axes of the fiber are not aligned with respect to the applied field in a direction that produces a suitable electro-optic effect. [0048] An improved optical fiber poling apparatus 10 is shown in [0049] Referring to [0050] To make a high voltage sensor using the sensitized shaped fiber, the fiber may be unrolled from the take up roll 30 ( [0051] The shaped optical fibers and the corresponding groove in the sensor insulator provide enhanced alignment accuracy of the principal electro-optic axes of the fiber with the electric field to be measured, which provides a more accurate voltage sensor. In addition, the shaped optical fibers may be made with a wide variety of very highly birefringent core/cladding designs. [0052] Recent work by Fujiwara et al., [0053] In another embodiment, application of UV radiation to change the refractive index in an optical fiber core is a method for creating various devices including Bragg gratings or long period gratings. Generally, Bragg gratings are constructed by placing the fiber at the intersection of two UV beams, whose angle of intersection can vary depending upon the type of device. The intersection region at the fiber has different properties which will affect the device created. These properties include an intensity pattern, a UV polarization direction, and the bisector of the UV beam propagation directions. This invention utilizes the mechanical registration of the birefringent fiber to align, at some predetermined angles, the birefringent axis with any or all of the axes described by these properties. The mechanical registration can also serve as an external reference for the orientation of the intensity pattern written into the grating by the UV exposure (in this case the fiber birefringence need not be relevant). [0054] As an example, a chirped fiber Bragg grating (like one used for dispersion compensation) used in reflection will have a net birefringence which is proportional to B·D·λ, where D is the dispersion (in ps/nm), λ is the wavelength, and B is the internal birefringence. The internal birefringence is defined as the birefringence of the fiber due to construction and/or UV exposure and/or any other causes. To reduce this net birefringence, it is possible to splice an additional birefringent fiber to the front of the grating, or to write the grating into a birefringent fiber. In the former case, the splicing must be done to align the additional birefringent fiber with the birefringence of the grating. In the latter case, the UV induced birefringence must be along either the slow or fast axis of the fiber. Both of these processes require knowledge of the principal birefringence axes of the fiber, which can easily be visually identified using the shaped birefringent optical fibers described in the '187 and '448 applications. Once the principal axes are identified, they may be easily aligned with the UV polarization and/or propagation direction. [0055] Another example is the intentional alignment of UV induced birefringence with the axis of a birefringent fiber in order to retain the polarization maintaining properties of the device. Without such alignment, the total birefringence (direction and/or magnitude) can change as a function of length along the fiber, causing the output polarization state to rotate as wavelength changes. This will degrade the performance of any polarization sensitive devices subsequent to this device, as well as the operation of the device itself. [0056] Referring to [0057] Properly configured platens may be used with shaped birefringent optical fibers to make a wide variety of devices with Bragg gratings or long period gratings. For example, rocking filters are fiber devices in which long-period gratings are used to convert light oriented along one principal axis of a birefringent fiber to light oriented along the other principal axis. Polarization conversion occurs when the wavevector corresponding to the long period grating is equal to the difference between the wavevectors of light traveling on the principal axes (the “fast” and “slow” or relatively lower and higher effective indices). Equivalently, the spatial period of the grating is equal to the beat length of the birefringent fiber. The long period grating can be generated by various means, including by imposing an external periodic stress on the fiber, or more conventionally, by writing a photoinduced periodic refractive index pattern in the fiber using a series of localized UV exposures spaced along the fiber. Applications of rocking filters include wavelength filtering and control of differential polarization delay for polarization mode dispersion compensators. In this last application, rocking filters transfer signals between the fast and slow axes of propagation in sections of PM fiber in a controlled way, thus adjusting the relative time delay between the two signals. [0058] In order for photorefractive rocking filters to achieve efficient conversion between polarization states, the grating must be written with the UV light incident at 45 degrees from the birefringent axes of the fiber. Since rocking filters can be several centimeters in length, maintaining accurate orientation of the fiber with respect to the light over the entire grating length can be difficult. With normal birefringent fiber, this is difficult because the exterior of the fiber is round, and there is no feature for observing the orientation of the fiber, or for holding it in the proper orientation. Conventional approaches to the problem require observing the cleaved end of the fiber to locate the internal fiber structures that induce the birefringence, and then attempting to orient these structures in the writing fixture, and maintaining the orientation during writing. This approach is tedious, time consuming, and can result in poor alignment accuracy that degrades the performance of the rocking filter. Therefore, improved means of initially orienting the fiber, and maintaining the orientation is needed. [0059] Referring to [0060] Shaped optical fibers may be used to advantage in aspects of packaging optical fiber Bragg gratings. Gratings are typically written in a short length of an optical fiber and are wavelength tuned by linearly stretching the fiber by pulling on its ends, or by otherwise straining the fiber. For example, as shown in [0061] When a grating is written, a small birefringence is induced, whose orientation is determined by the write direction. Having such a grating written in a shaped fiber allows this induced birefringence to be registered to an external feature of the fiber throughout its entire length. Furthermore, in the case of a PM fiber, the induced birefringence will also be registered to the internal birefringence axes of the fiber. Referring to [0062] In another embodiment, a shaped fiber wrapped around an appropriately configured expandable mandrel and encased in a potting material, such as a UV or chemically curable, thermoset or thermoplastic material, such as, for example, a curable epoxy adhesive, can be used for polarization rotation. If the fiber is standard single mode fiber, such a procedure results in a slight birefringence due to bending of the fiber. Aligning the angle of the induced or permanent polarization axis (for PM fiber) of the fiber precisely with respect to the expandable mandrel can greatly improve the ability to control the polarization since the stress will be applied in a uniform direction along the whole length of fiber with respect to any internal birefringence. Encasing the fiber in epoxy actually allows for a magnification of the effects of the expandable mandrel since a large effective squeezing force is experienced by the fiber as the expanding actuator presses it against the epoxy potting. This arrangement can be used to reduce the amount of expansion necessary to achieve polarization rotation (this can also be applied to the case where a Bragg grating is stretched since the squeezing affords an index change, which may be equivalent in many cases to a length change in functionality; for example for tunable Bragg grating applications). In this case (epoxy encapsulation), birefringent axes are most desirably registered to the mandrel since any twist of the fiber combined with the large stress modulation over the length of the fiber (typically a meter or more) may result in unwanted polarization mode coupling. [0063] In another Bragg grating application, shaped optical fibers may be used to identify the alignment direction of a blazed grating. Typically, fiber Bragg gratings are made so that the grating fringes are as perpendicular as possible to the fiber's longitudinal axis, but some applications, such as side-tapping and reflection-reduction, require the grating fringes to be written into the fiber with an angle to the longitudinal fiber axis. By tilting, or “blazing”, the grating, the coupling between the core mode and radiation modes is enhanced, so these gratings are also referred to as radiation-mode couplers. [0064] A tilted grating can be used as a light tap, where a portion of the light in the fiber core is routed out of the fiber for subsequent analysis. In strongly tilted gratings, i.e. gratings with a large angle with respect to the direction of light travel down the length of the optical fiber, the light coupled into radiation modes by the tilted grating is strongly polarized, allowing researchers to make in-line polarimeters that may be used in polarization mode dispersion (PMD) compensator applications and polarization interleavers. [0065] Polarimeters have been made by fabricating a set of four tilted gratings oriented at various angles with respect to one another into the fiber, as shown in [0066] The tilted grating can also be chirped so that different wavelengths of light are emitted at different locations along the fiber length. A detector array, such as a charge coupled device (CCD), can be mounted onto the side of the fiber from where the light is emitted and the wavelengths of light traveling down the fiber can be detected and monitored. A device of this nature would be useful in dense wavelength division multiplexing (DWDM) telecommunications applications, for example to monitor the strength and wavelength of communications channels propagating down the fiber. As with the polarimeter, one must launch light into the fiber and detect where the light is emitted to mount the detectors. Shaped fibers can eliminate these complicated steps in a manufacturing process and reduce the device costs. [0067] In yet another embodiment, optical fiber Bragg gratings may also be written in a polarization-maintaining (PM) fiber for use in polarization optical delay lines. Polarization delay lines are an integral component of polarization mode dispersion (PMD) compensators. An optical signal in a network system accumulates PMD as a result of traveling through the optical fiber and optical components. It will acquire a differential delay between the two orthogonal states of polarization supported by the fiber. This delay results in signal dispersion, and if severe, an inability to determine the information content of the signal. [0068] The delay between the orthogonal polarization states may be reduced with two chirped gratings written in a PM fiber. Each of the two orthogonal polarization states is sent down one of the fibers and is reflected from the chirped grating. By tuning one of the gratings with respect to the other, the reflection point in the grating changes, and the light travels a shorter path in one of the legs. By correctly delaying the polarization that was leading in the optical signal, the signal can be recombined after reflecting from the gratings, thus reducing or eliminating the differential delay. [0069] Using PM fiber in the polarization delay line has an advantage over standard single-mode (SM) fiber. If the signal is launched correctly into the PM fiber, it will maintain its state of polarization upon reflection from the chirped grating, and thus can be recombined with the orthogonal polarization reflected in the other grating using a polarization beamsplitter. A polarization beamsplitter, also called a polarization splitter or combiner, allows one beam to be split into two orthogonal polarization components or, when operated in reverse, allows two oncoming orthogonal polarization component beams to be combined into one beam. Having a shaped outer surface or feature on the fiber aids in writing the grating in the fiber, as a more uniform grating provides better performance. [0070] PM fiber can also be used in another component of a PMD compensator, a polarization transformer, which transforms and aligns the two orthogonal states of polarization supported in the fiber to the polarization delay line. Multiple polarization rotators may be combined to create this polarization transformation. In one embodiment, each rotator is made by winding many loops of PM fiber onto a piezo-electric actuated expandable cylinder (See, for example, [0071] The shaped fibers may be used in a wide variety of optical fiber devices in which multiple birefringent fibers and/or multiple Bragg gratings in the fibers are oriented with respect to one another. For example, fused fiber polarization splitters (and equivalently, polarization combiners) are key components of many important fiber optic systems including pump combiners for Er-doped and Raman fiber amplifiers, polarization mode dispersion compensation systems, and a variety of fiber sensors. These components may be fabricated by fusing two birefringent (typically polarization-maintaining (PM)) fibers together with two surfaces in contact, preferably in a side-by-side arrangement. In performing the fusion, it is necessary to control the orientation of the birefringent axes of the PM fibers while the fusing takes place, to obtain good polarization coupling and extinction. With normal PM fiber, this is difficult because the exterior of the fiber is round, and there is no feature for observing the orientation of the fiber, or for holding it in the proper orientation. Conventional approaches to the problem require observing the cleaved end of the fiber to locate the internal fiber structures that induce the birefringence, and then visually orienting these structures in the fusion fixture, which is meant to maintain the orientation during fusion. This approach is tedious, time consuming, and can result in poor alignment accuracy that degrades the performance of the coupler. [0072] Referring to [0073] A process very similar to that described above and shown in [0074] A polarization-maintaining coupler as described in the paragraph above can be used to fabricate another optical device known as a depolarizer, as disclosed in U.S. Pat. No. 5,218,652 and the related technical article by Lutz, IEEE Photonics Technology Letters, Vol. 4, No. 4, April 1993, pp. 463-465. One purpose of this device is to take a (preferably constant intensity) input of any random state of polarization and convert it to an output that is completely unpolarized. One embodiment of such a depolarizer device is made from a PM coupler having two PM input fiber ends (e.g., A and B), two PM output fiber ends, and a coupling ratio such that at least ⅔ of the light entering input A will exit a particular output, e.g., D. Output D is then optically connected to input B to form a recursive loop. The degree of depolarization can then be tuned by adjusting the angles between the polarization axes of fibers D and B in the loop, as indicated in [0075] In another aspect, in a twin core fiber it is difficult to discern the proper orientation of the fiber. In every operation using twin core fiber, an initial orientation step is required, which greatly increases production time and costs. This orientation step makes it difficult and time consuming to write a Bragg grating into one or both cores, to selectively irradiate one core with light, or to splice the twin core fiber to a standard single mode fiber. Referring to [0076] Rapid core orientation would greatly reduce the costs of many devices made using twin core fibers. For example, a coupler may be made in a twin core fiber by heating a section, typically with a laser, and then simultaneously writing a linear or non-linear chirped Bragg grating in both cores. This Michelson interferometer device, which does not require a circulator, provides low cost signal dispersion compensation and saves about 1.5 dB of insertion loss. A similar device could be used as a dispersion clean-up device at a receiver or as a PMD compensation variable delay line. In addition, Mach-Zehnder interferometer devices could be manufactured by making two couplers along a length of fiber, then trimming using UV or localized heat to adjust one of the interferometer legs, or by simultaneously writing a Bragg grating into both cores before the couplers are made, and then making the couplers using localized heat treatment. Further, frustrated coupler devices could be used by, for example, UV irradiating one fiber and writing a Bragg grating into another to make an add-drop coupler. Optical switches may be made by polishing down into the fiber to access the evanescent fields of one or both fiber cores in the twin core fiber. A material such as, for example, LiNbO3, may be applied against the region that accesses the modes in the cores and controls the coupling of energy from one core to another. Alternatively, a localized heat source along a coupler in the twin core fiber could be used to thermally control coupling from one core to another. [0077] Shaped fibers, both birefringent and non-birefringent, are also useful in any application where a location of an external feature of the fiber must be determined with accuracy. As noted above, both single mode (non-birefringent) and PM/PZ fibers may include lensed tips to couple with a light emitting device. The lenses may or may not be rotationally symmetrical. [0078] For example, referring to [0079] If the wedge-shaped lens tip is formed on a PM or a PZ fiber, then the efficiency of the coupling depends on two alignment steps. First, the leading edge 510 of the wedge-shaped lens must be rotationally aligned with the internal birefringent axes of the fiber, and then the surfaces of the light emitting device must be rotationally aligned with the line formed by the intersecting faces of the lens, referred to herein as the lens axis. Even if the lens on the PM or PZ fiber is rotationally symmetrical (for example, a conical lens), the internal birefringent axes of the fiber must still be aligned with the axes of the light emitted from the device. The elliptical output beam may also be polarized along the axes of the beam ellipse. [0080] Referring to [0081] Referring to FIGS. 12A-C, an alignment device 550 may be configured with a longitudinal V-shaped groove 551 designed to accept the V-shaped cross section of the fiber 520 of [0082] To align a standard single mode fiber (circular cross-sectional shape, non-birefringent) with a light emitting device, a short piece of the shaped fiber 520, which is shown in [0083] The alignment device 550, which includes the longitudinal V-shaped groove 551, may be used as shown in FIGS. 12A-D to align optical fibers to light emitting devices, or, to align fibers to one another. The groove 551 limits the side-to-side movement of the fiber during the alignment procedure, and provides a rotational alignment function as well. In addition, the alignment groove may be used, along with the shape and size of the shaped fibers, to facilitate alignment of fibers with different diameters and core/cladding regions. For example, a standard 125 micron fiber with a unique core design or shape, such as a Panda fiber, could be placed in abutting relationship and aligned with a specially shaped optical fiber in the V-groove 551 of the alignment device 550 (See [0084] Referring to [0085] In another embodiment shown in [0086] A number of embodiments of the invention have been described. Nevertheless, it will be understood that various modifications may be made without departing from the spirit and scope of the invention. Accordingly, other embodiments are within the scope of the following claims. Optical devices using shaped optical fibers and methods for using shaped optical fibers. 1. A method for sensitizing an optical fiber, comprising: (a) providing a section of a shaped optical fiber having a waveguiding region with a known internal geometry, wherein the waveguiding region is oriented at a known angle with respect to one of: (i) a portion of an outer surface of the fiber, and (ii) one or more features on the outer surface of the fiber; and (b) passing the fiber between a first electrode and a second electrode such that an electric field is applied to the waveguiding region, wherein at least one of the first and second electrodes have a feature that engages at least one of the portion of the outer surface of the fiber and the feature of the fiber to maintain the orientation of the waveguiding region of the optical fiber with respect to the applied electric field. 2. The method of 3. The method of 4. The method of 5. The method of 6. A method for making an electric field sensor, comprising: (a) providing a shaped optical fiber having a waveguiding region with transverse axes, wherein the axes are oriented at a known angle with respect to one of: (i) a portion of an outer surface of the fiber, and (ii) one or more features on the outer surface of the fiber; (b) poling the fiber; and (c) wrapping the fiber about a cylindrical insulator, wherein an outer surface of the insulator comprises a feature that engages at least one of the portion of the outer surface of the fiber and the feature of the fiber to maintain the orientation of the waveguiding region of the optical fiber with respect to an applied electric field, and (d), applying an electric field to the optical fiber to produce electro-optic axes exhibiting a phase difference in light transmitted through the optical fiber, wherein the phase difference has a known orientation with respect to the transverse axes of the fiber. 7. The method of 8. An electrical voltage sensor comprising a birefringent optical fiber, wherein the fiber has a non-circular cross sectional shape. 9. The sensor of 10. The sensor of 11. A method for making an optical fiber Bragg grating, comprising providing an orientation device having a surface feature; inserting into the surface feature of the orientation device a shaped optical fiber having a waveguiding region with transverse axes, wherein the axes are oriented at a known angle with respect to one of: (i) a portion of an outer surface of the fiber, and (ii) one or more features on the outer surface of the fiber; and writing a Bragg grating in the waveguiding region at a known angle with respect to the transverse axes of the waveguiding region. 12. The method of 13. The method of 14. The method of 15. The method of 16. A method for altering the birefringence of an optical fiber, comprising: (a) providing a shaped optical fiber having a waveguiding region with transverse axes, wherein the axes are oriented at a known angle with respect to one of: (i) a portion of an outer surface of the fiber, and (ii) one or more features on the outer surface of the fiber, (b) straining the optical fiber in a device having a surface with a feature that engages at least one of the portion of the outer surface of the fiber and the feature of the fiber to maintain the orientation of the waveguiding region of the fiber with respect to the device. 17. The method of 18. The method of 19. The method of 20. The method of 21. The method of 22. A method for making a polarization splitter or combiner, comprising: (a) providing a first shaped birefringent optical fiber with a first surface feature having a known orientation with respect to the principal axes of the first fiber; (b) providing a second shaped birefringent optical fiber with a second surface feature having a known orientation with respect to the principal axes of the second fiber; and (c) fusing the first fiber and the second fiber together in an arrangement such that the principal axes of the first fiber are aligned at a known angle with respect to the principal axes of the second fiber. 23. A method for making a polarization maintaining coupler, comprising: (a) providing a first alignment fixture with a first alignment feature; (b) providing a second alignment fixture with a second alignment feature; (c) mounting in the first alignment feature a first surface feature of a first shaped birefringent optical fiber, wherein the first surface feature has a known orientation with respect to the principal axes of the first fiber; (d) mounting in the second alignment feature a second surface feature of a second shaped birefringent optical fiber, wherein the second surface feature has a known orientation with respect to the principal axes of the second fiber; and (e) fusing the first fiber and the second fiber together such that the principal axes of the first fiber are aligned at a known angle with respect to the principal axes of the second fiber. 24. A twin-core optical fiber having a first core and a second core, wherein the fiber has at least one of a cross-sectional shape and a surface feature oriented at a known angle with respect to a line between the first core and the second core. 25. A birefringent optical fiber comprising a Bragg grating, wherein the fiber has a noncircular cross-sectional shape. 26. A polarimeter comprising the optical fiber of 27. A spectrum analyzer comprising the optical fiber of 28. A polarization dependent optical delay line comprising the optical fiber of 29. A device for stabilizing an optical amplifier pump laser, wherein the device comprises the optical fiber of 30. An optical fiber comprising an endface with a non-spherical lens having a lens axis transverse to the longitudinal axis of the fiber, wherein the lens axis has a known orientation with respect to at least one of: (i) an outer surface, and (ii) a surface feature of, the fiber. 31. The optical fiber of 32. The optical fiber as in 33. An optical interconnection between two optical fibers with dissimilar cross-sectional shapes, comprising:
(a) a first fiber having a first optical core, a first effective diameter, and a first endface with an essentially round outer surface; (b) a second fiber having a second optical core, a second effective diameter, and a second endface with a non-round outer surface; and (c) an alignment fixture having at least one continuous surface, wherein the first effective diameter and the second effective diameter are not substantially equal, and when the first and second fibers are placed in the alignment fixture with their respective endfaces in abutting relationship in contact with the continuous surface, the first and second optical cores are in optical communication. 34. The optical interconnection of CROSS-REFERENCE TO RELATED APPLICATIONS
TECHNICAL FIELD
BACKGROUND
SUMMARY
DESCRIPTION OF DRAWINGS
DETAILED DESCRIPTION
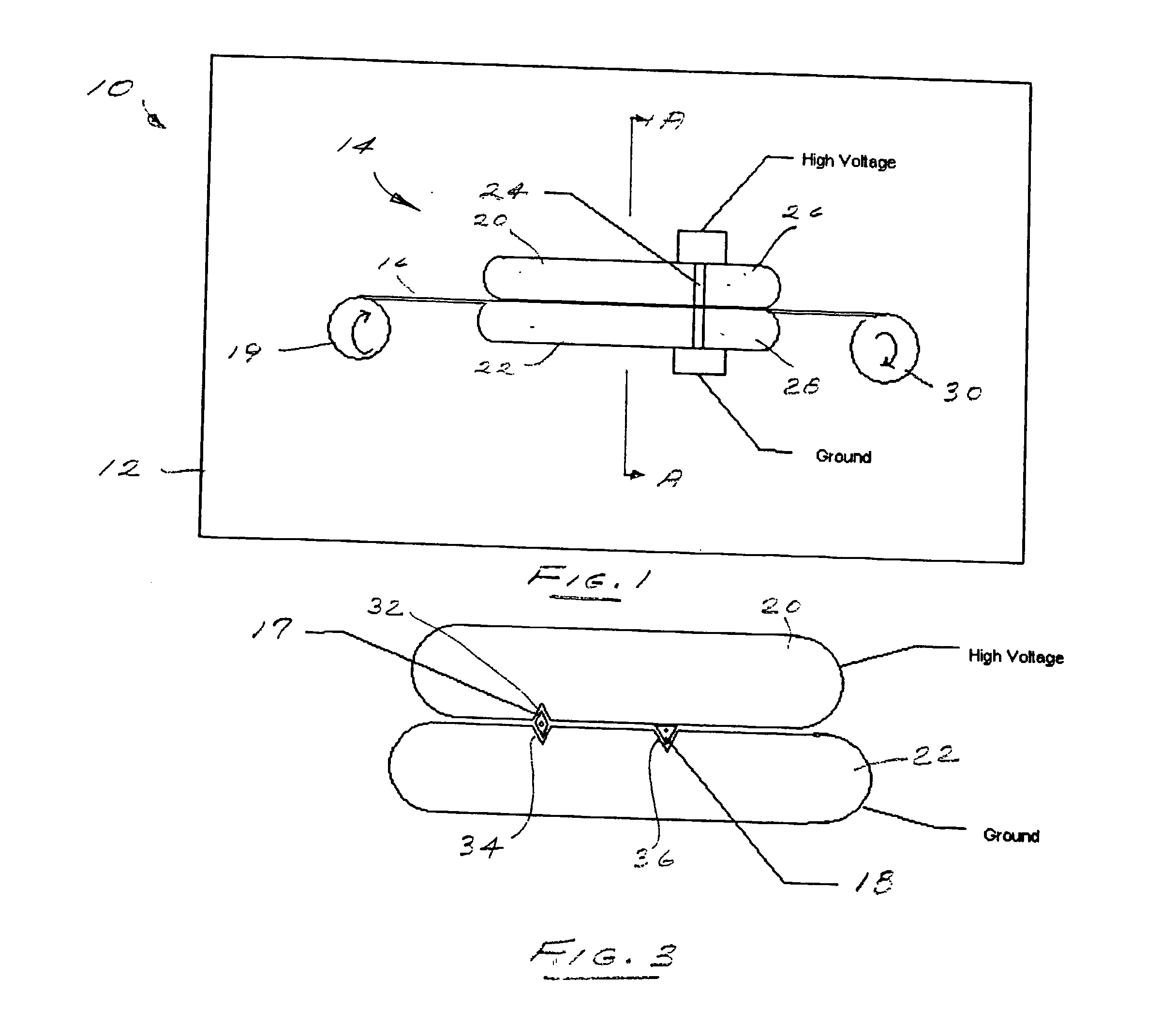
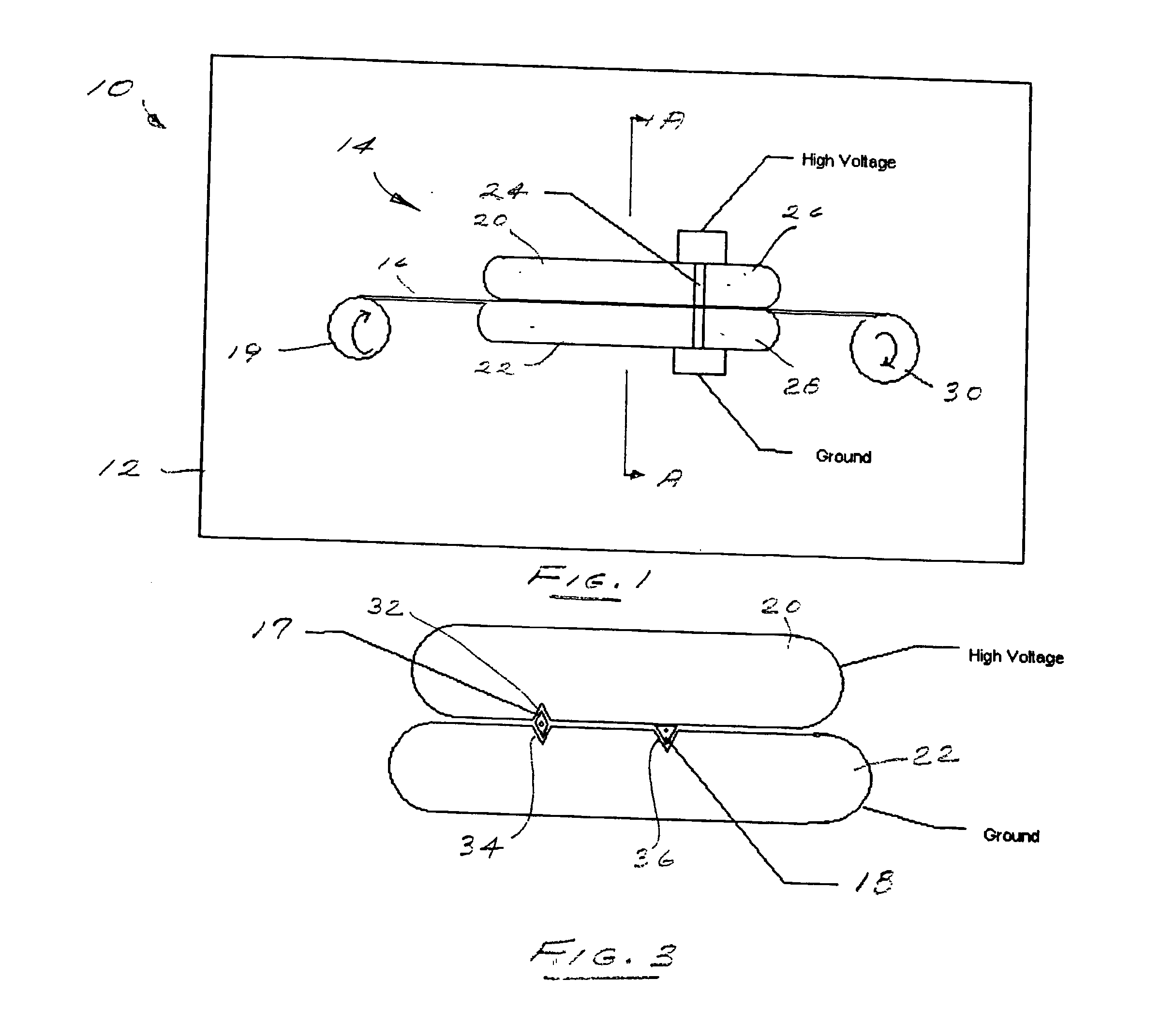
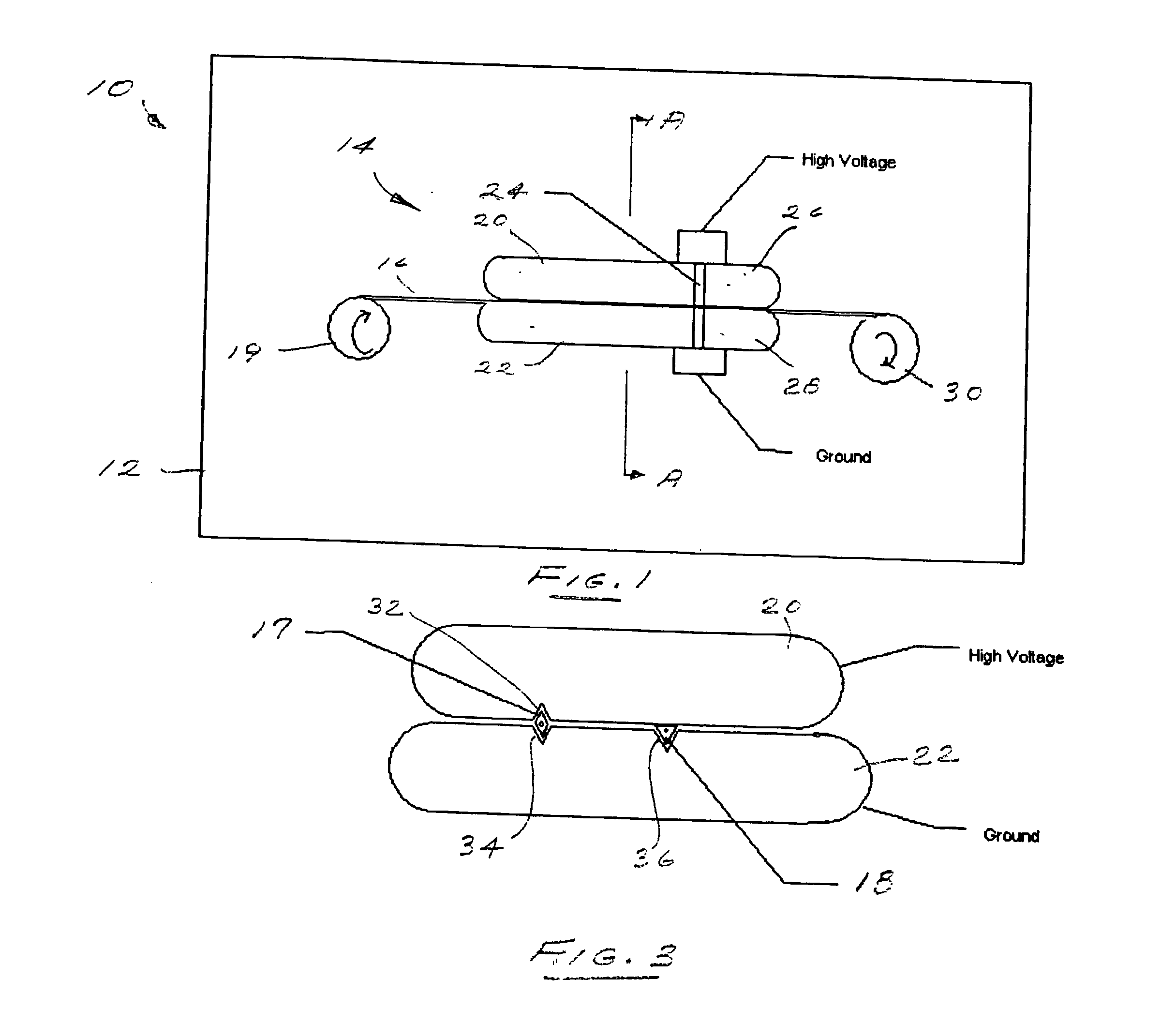
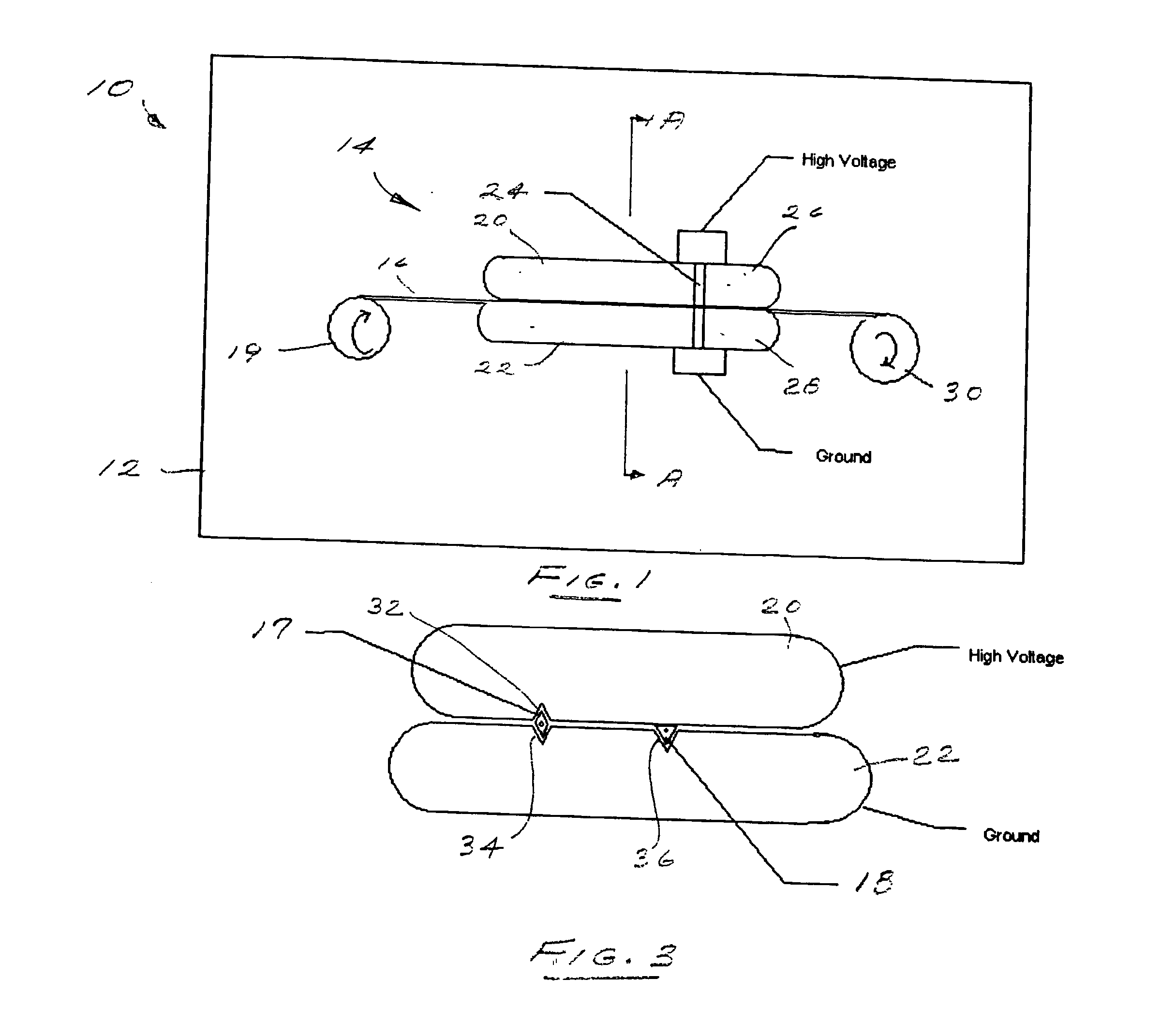
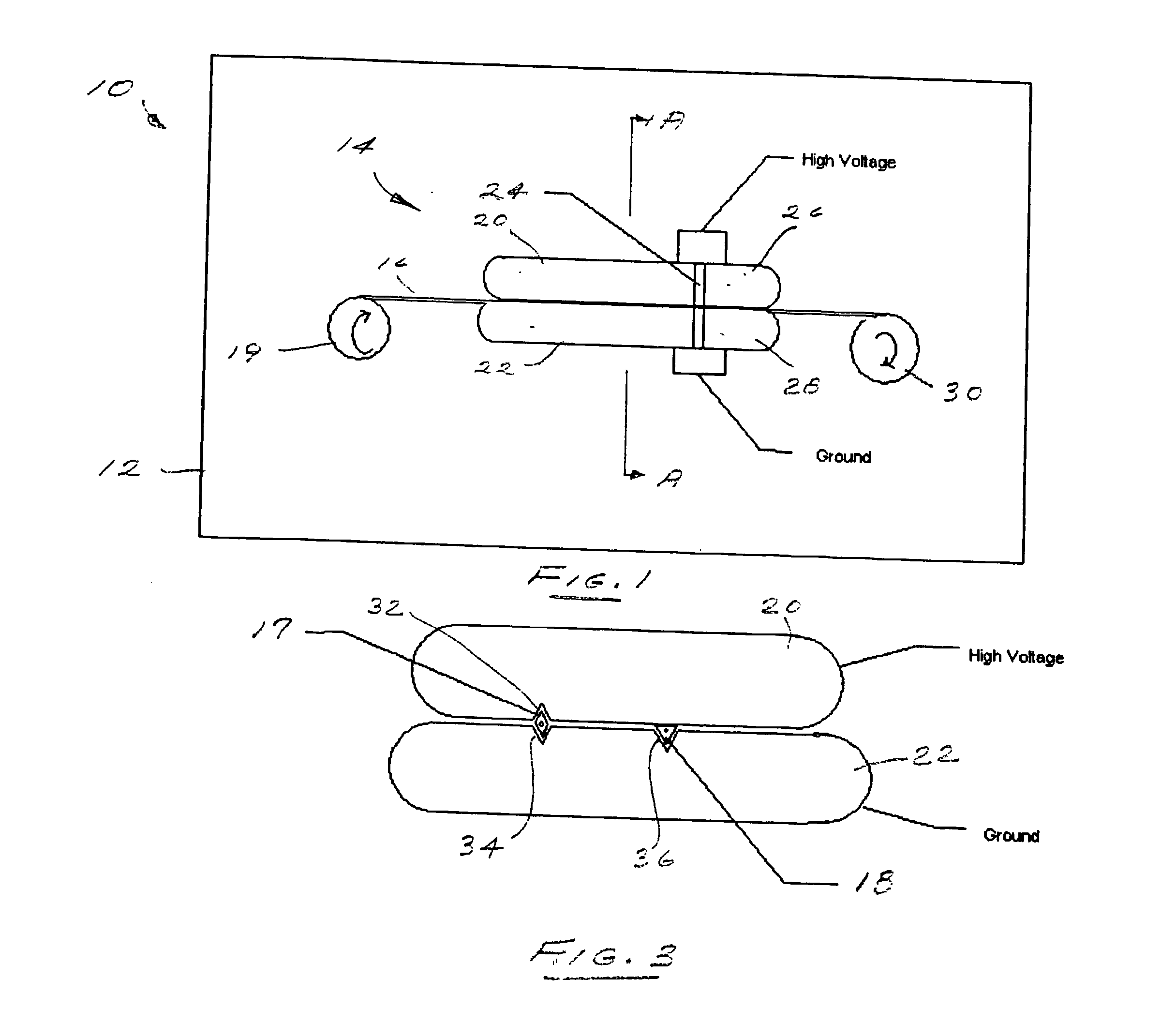
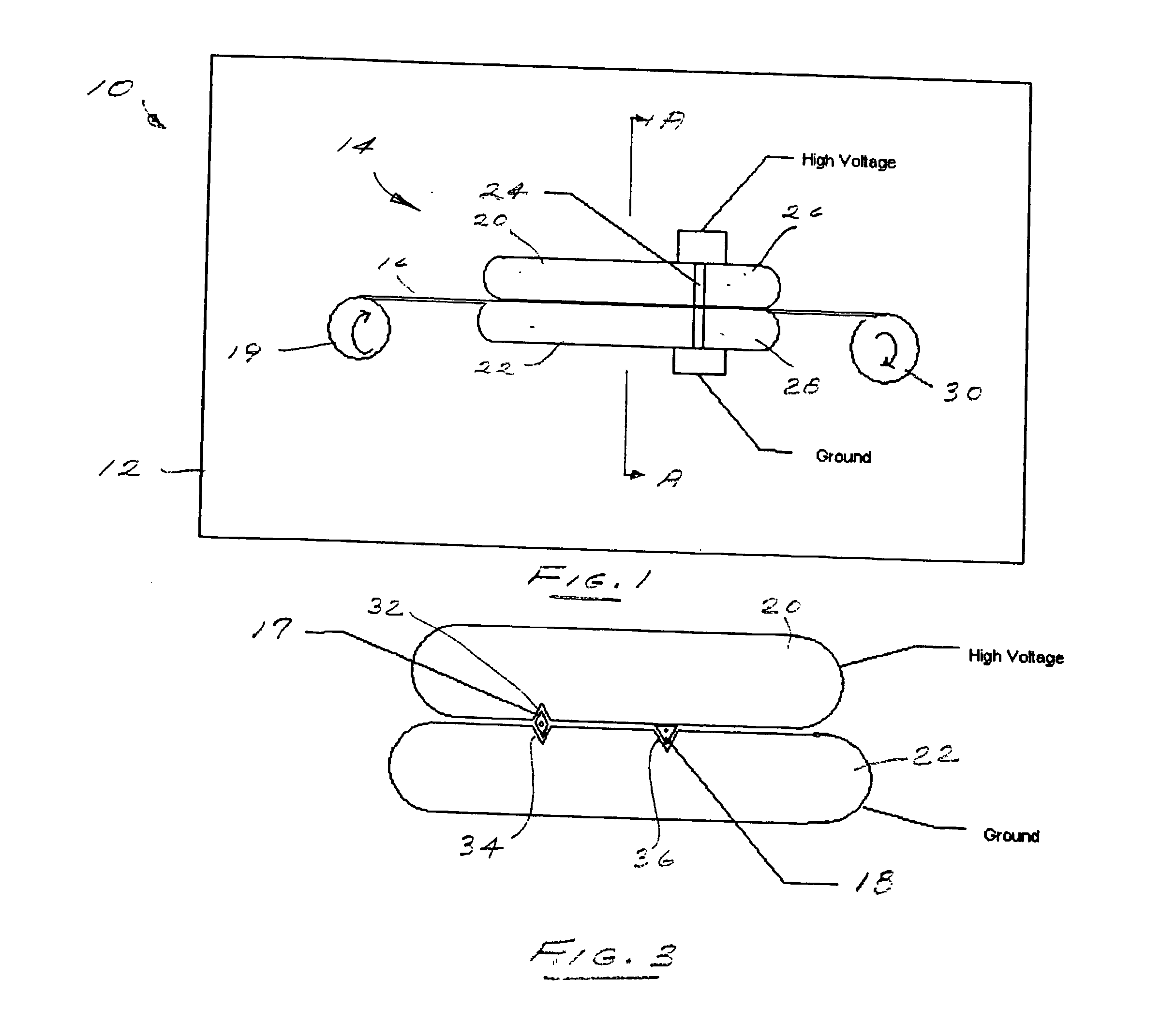
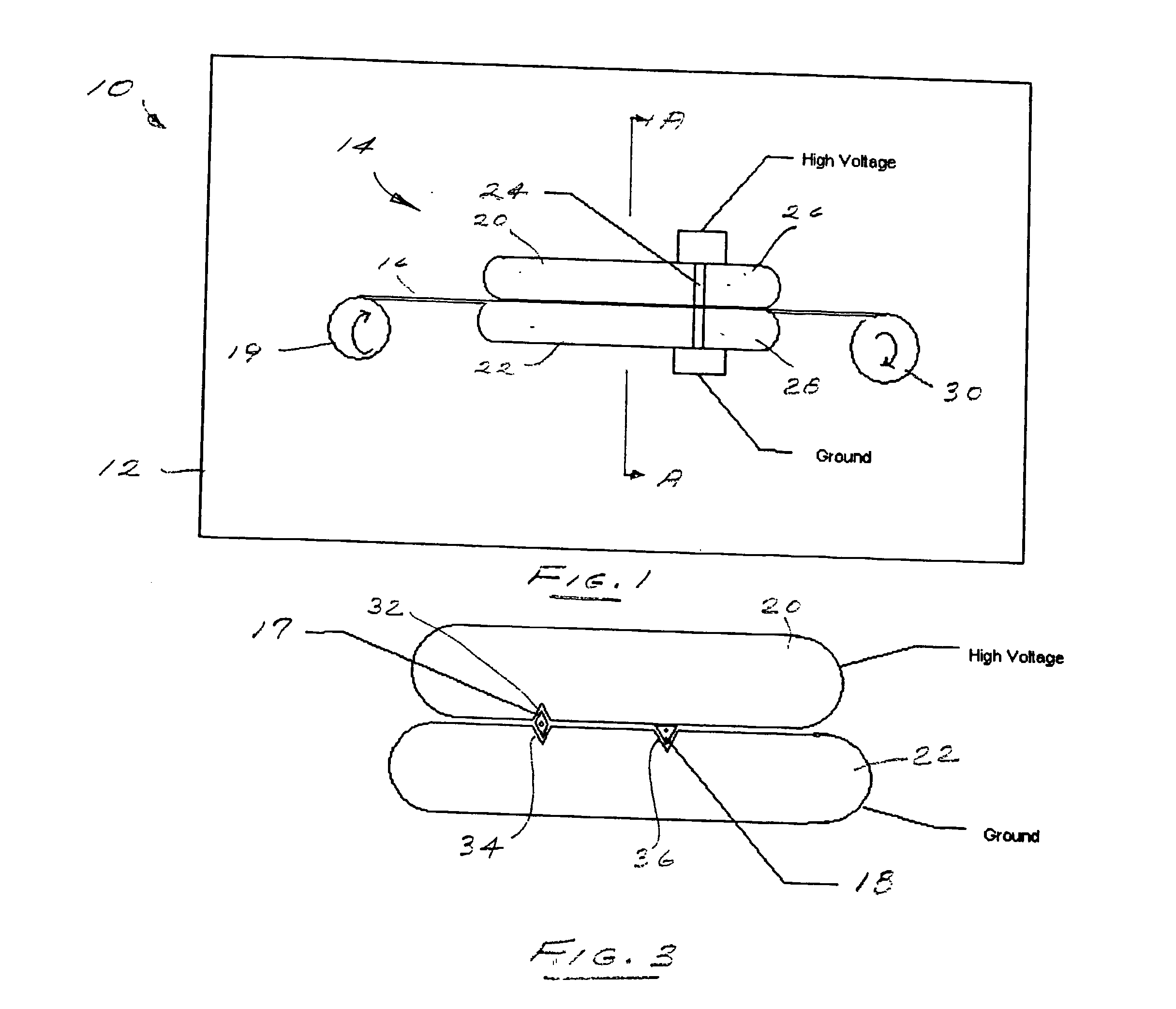
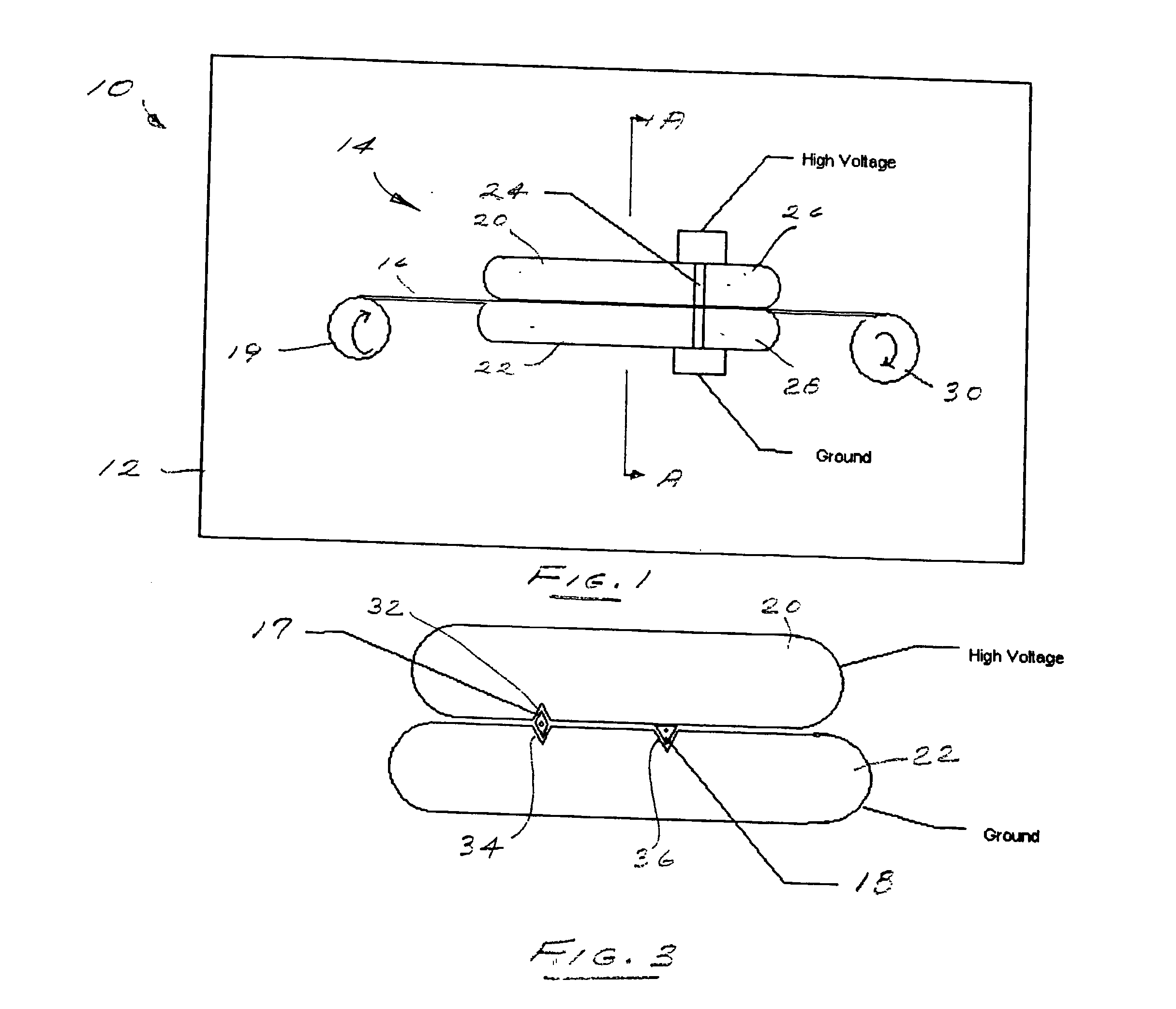
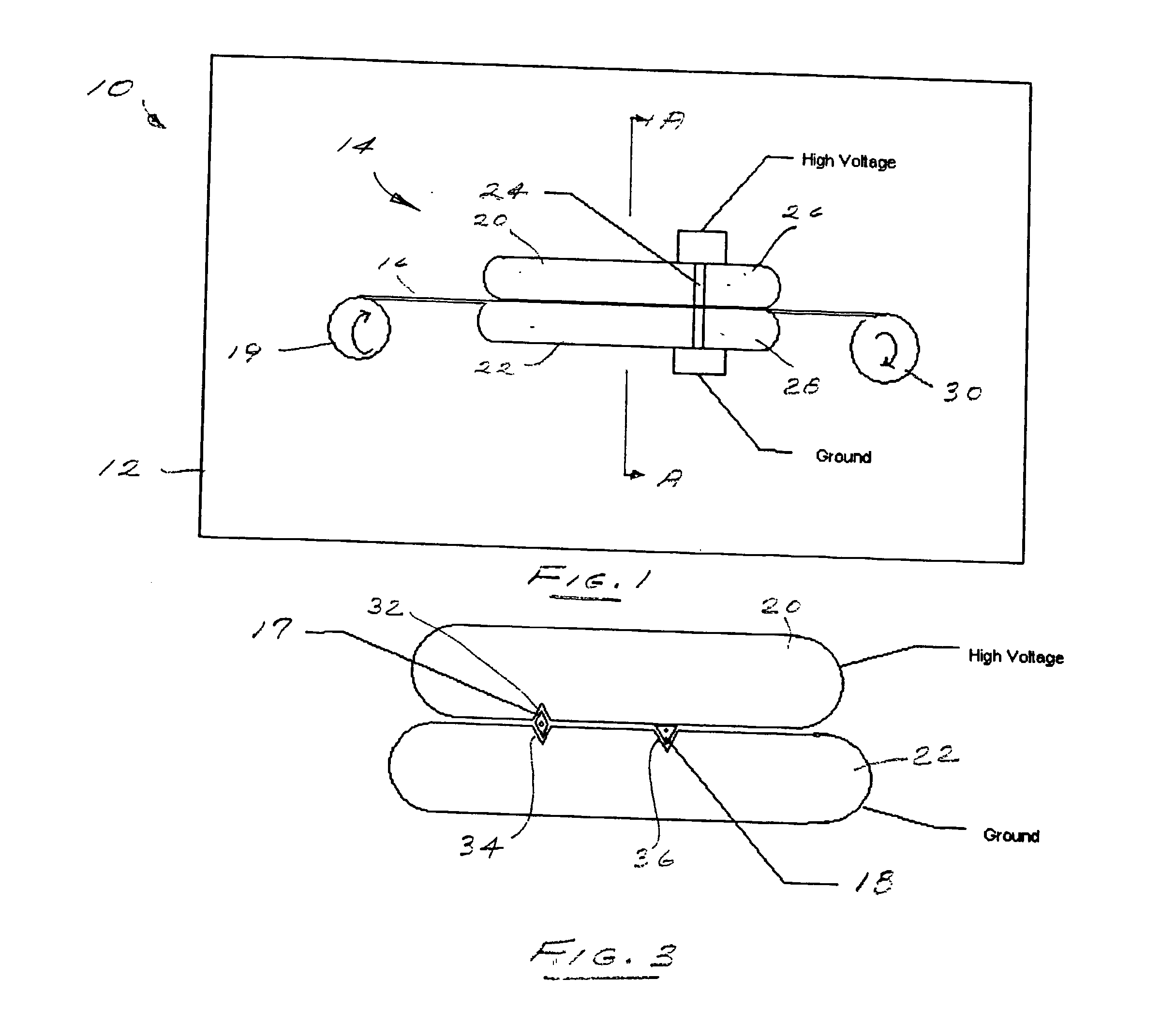
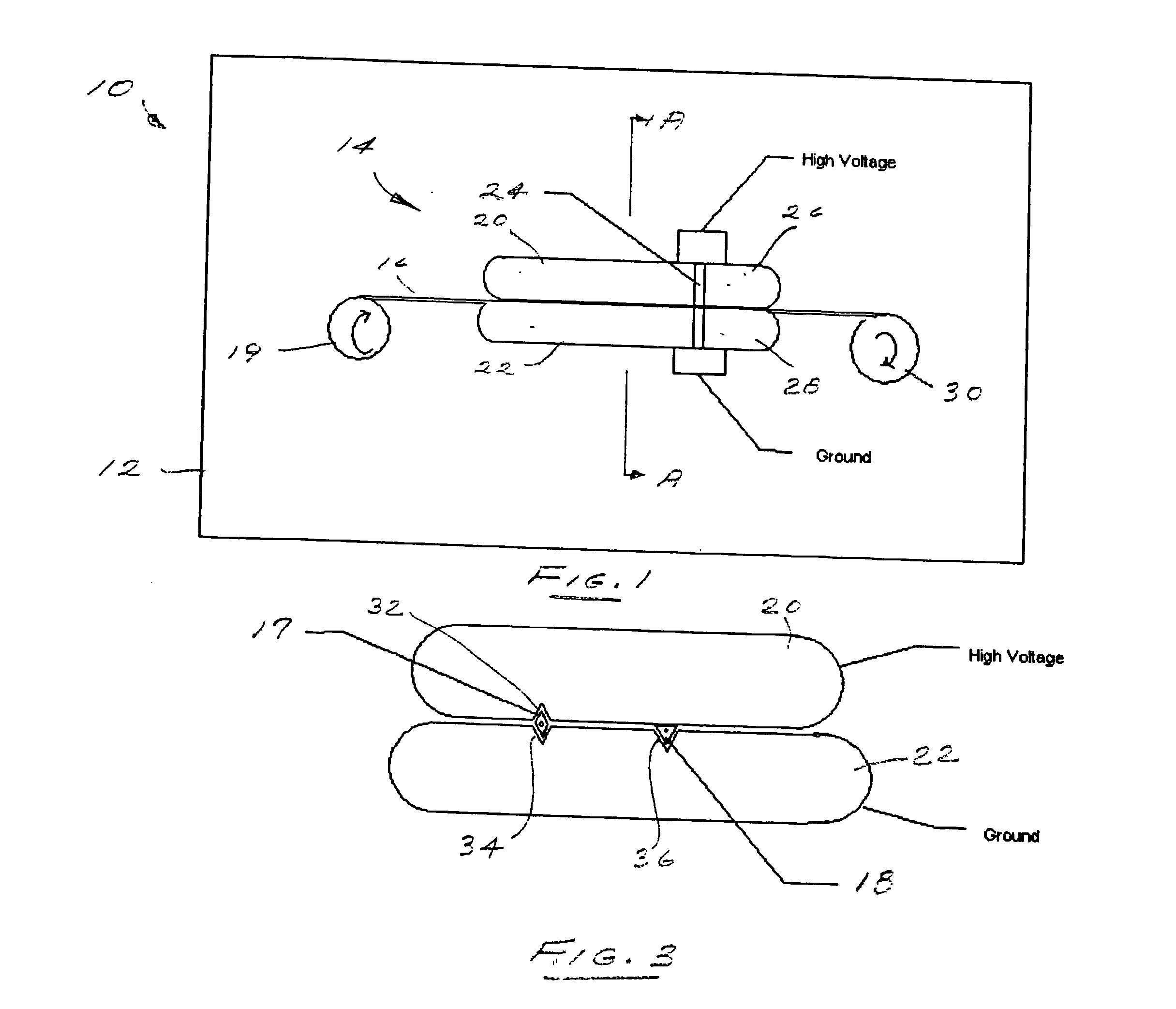
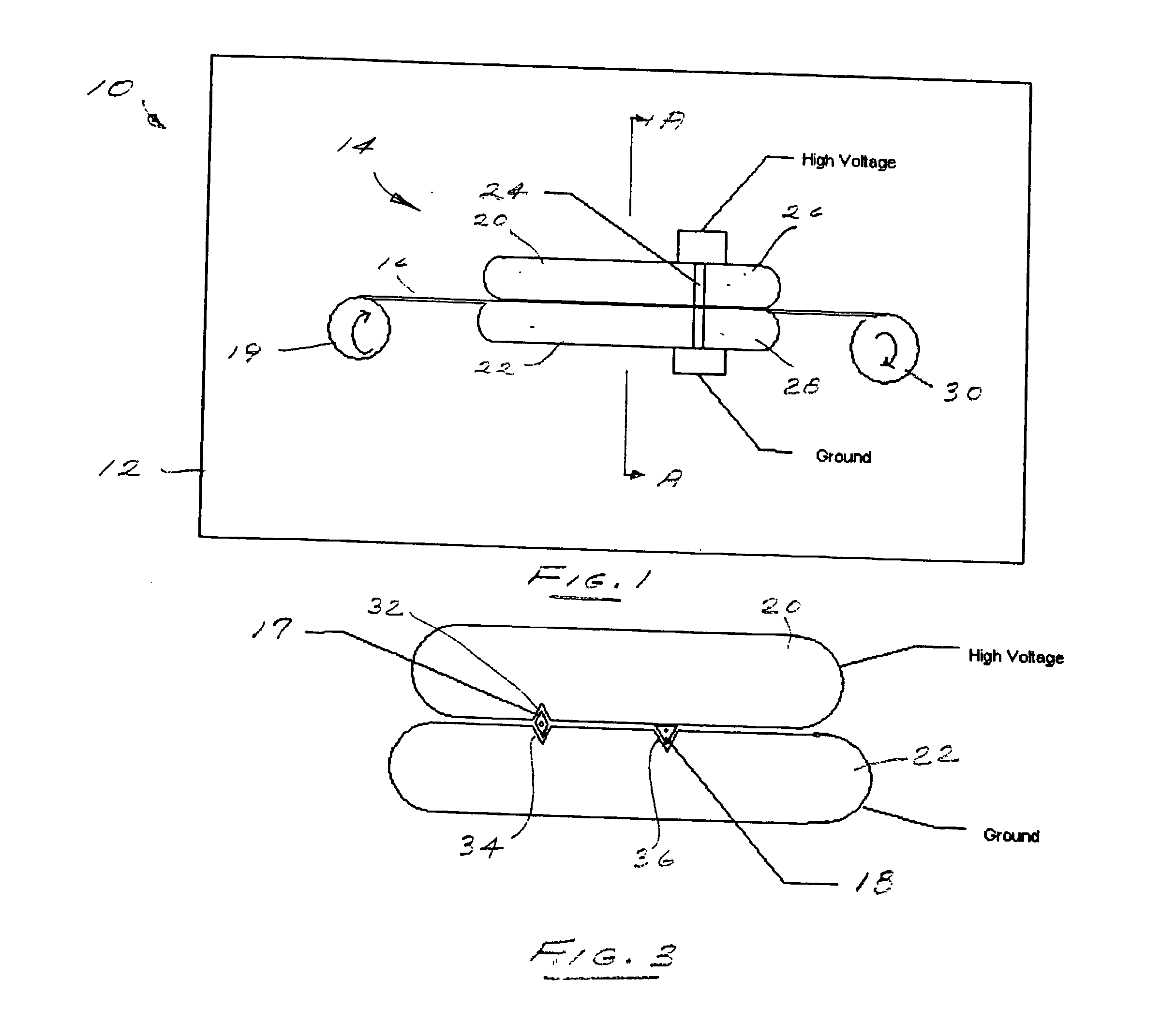
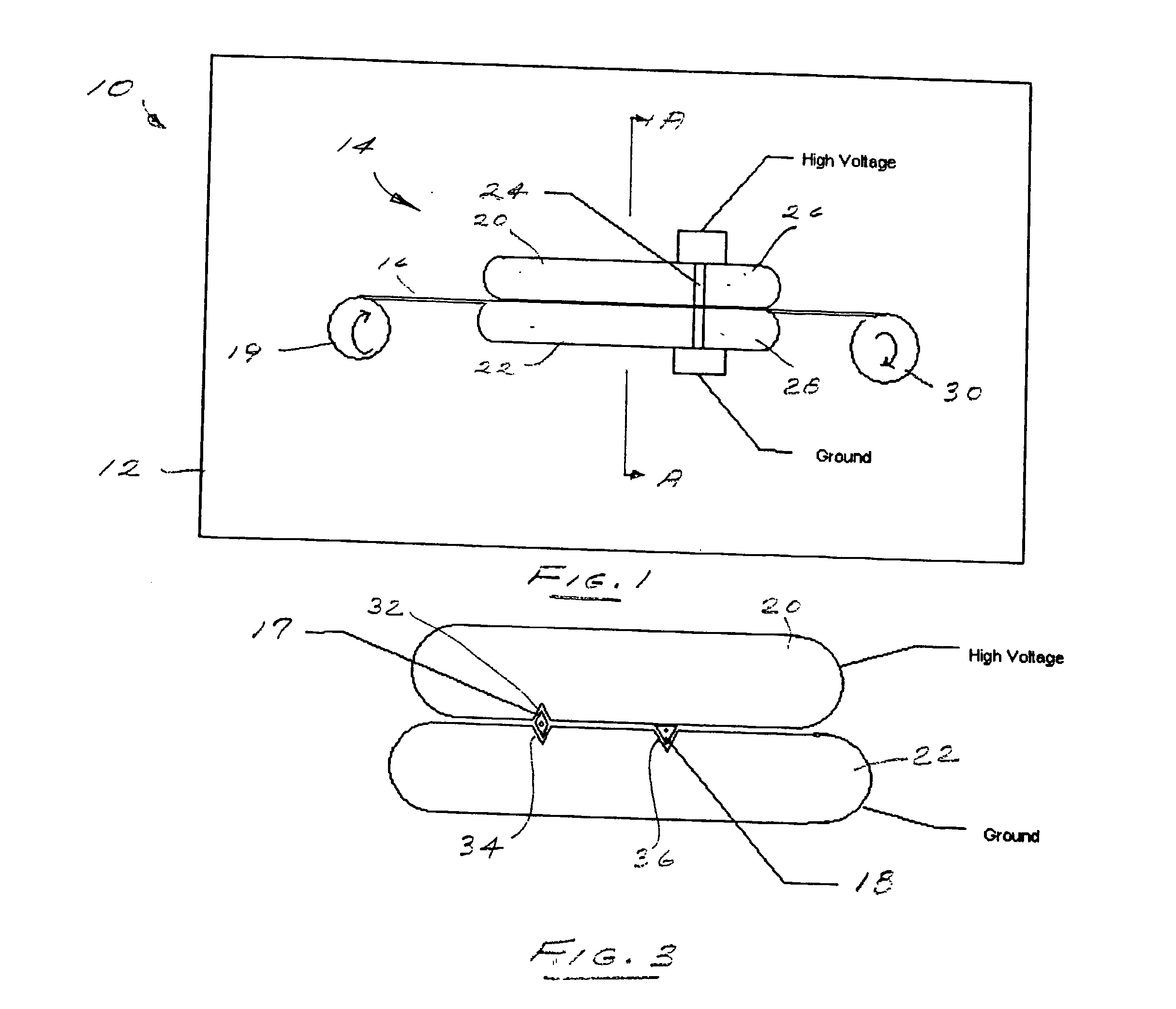
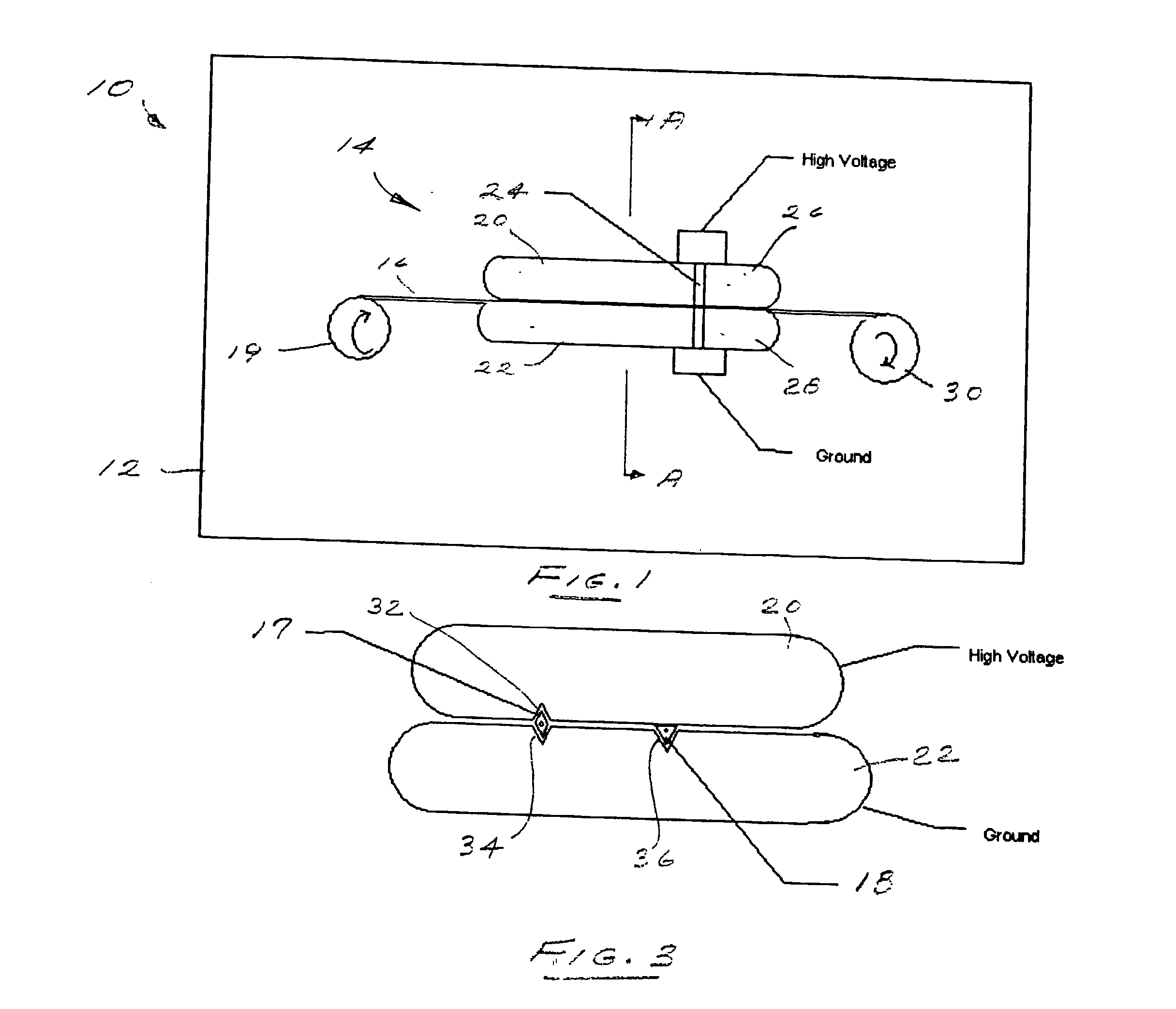
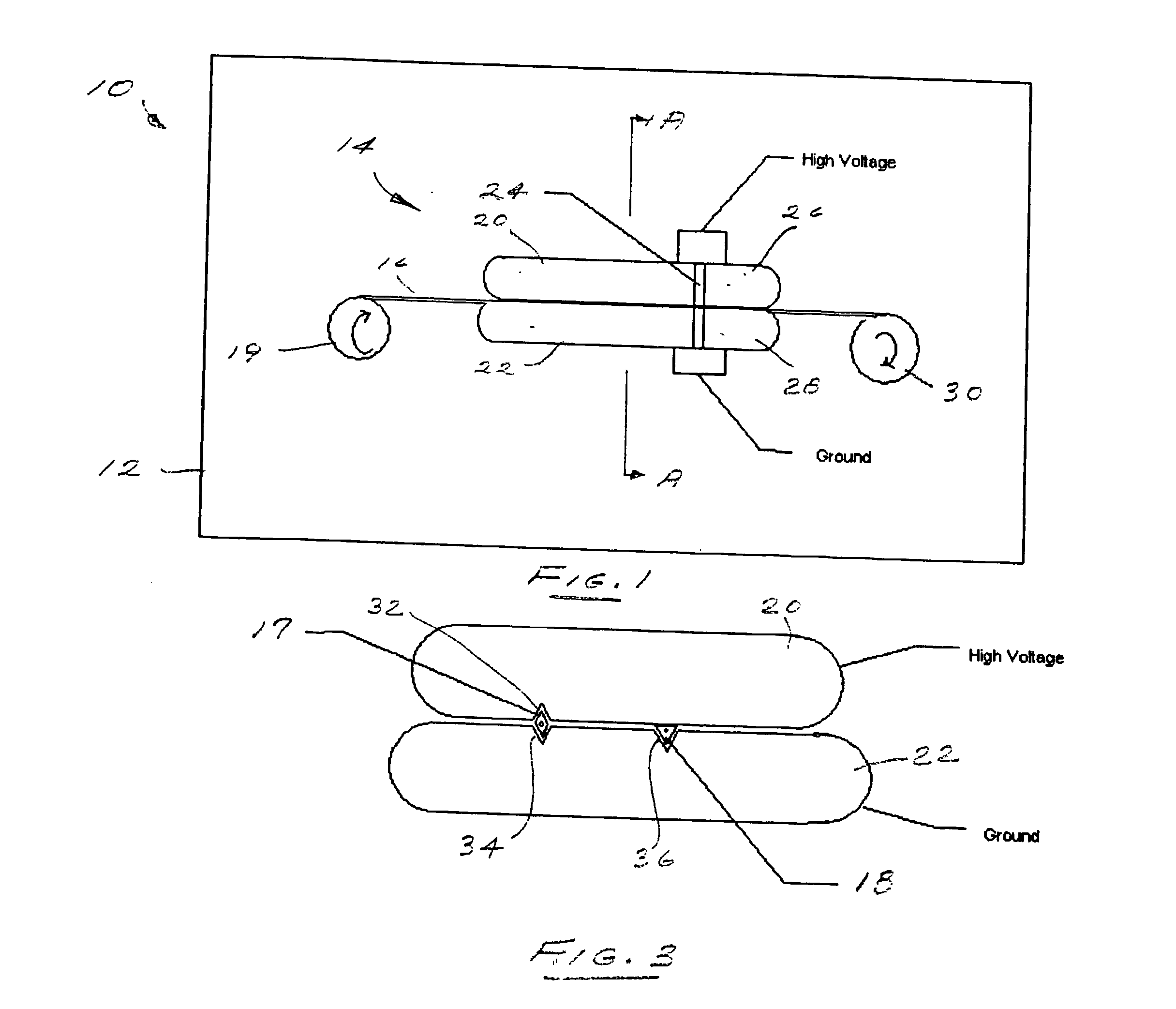