USE OF NANOPARTICLES FOR LABELLING OIL FIELD INJECTION WATERS
The field of this invention is that of the exploration and the exploitation of oil reservoirs. More precisely, this invention relates to the development of tracer fluids, more generally, that of aqueous liquids, intended to be injected under pressure in an oil reservoir, for example from an injection well up to a production well. The injected waters thus diffuse through the geological solid medium, which constitutes the oil reservoir, making it possible to study the latter by following the path of the injected waters. The goal is in particular to control the flows between the injection well(s) and the production well(s) and/or to evaluate the volumes of oil in reserve in the reservoir and, in fine, of optimizing the oil exploration and exploitation. It is well-known in the exploitation of an oil reservoir that, most of the time, no more than half of the oil initially present in the reservoir is extracted, or even less. The recovery by the primary means, that is, the use of extraction energy utilized from gases or liquids present in the subsoil and currently, initially, a certain pressure in the reservoir, allows for only extracting low percentages of the total oil present in the reservoir. To complete this primary recovery, one proceeds with a secondary recovery consisting in implementing what is called a “water drive” or “water flooding” production, that is, by injecting water in a well (injection well) at a location of the reservoir, so as to push the oil of the reservoir out of the subsoil, by at least one other called a “production well”. To improve the secondary recovery by water drive, it is known to add surfactants to the injection water. This technique for optimizing the oil recovery by pushing injected water, to which surfactants may or may not have been added, also involves tracers that can be easily detected in the liquid, namely the injection water and push water at the exit of production wells. These tracers allow for measuring the arrival time, that is, the time which passes between the injection of the injection and push waters in the injection well(s) and the moment when these injection water and push water charged in tracers come out at the exit of one of several isolated production wells. From this arrival time, one can determine the volume of the reservoir that constitutes the oil reservoir. This is one of the most important parameters which can be determined by the use of tracer fluids, since it allows, on the one hand, adjusting the quantity of surfactant introduced in the injection and push waters, and on the other hand, evaluating the additional quantity of oil that can be expected following the implementation of this optimized method for recovering oil. As long as the fluid containing the tracer has been detected at the production well(s), the method of study for analyzing, monitoring, and optimally recovering oil requires the concentration of tracers in the fluid produced at the exit to be measured, continuously or not, so as to be able to plot the curves of tracer concentration as a function of the time or as a function of the volume of fluid produced. Tracers in the injection water and push waters for oil reservoirs also enable detecting aberrations in the flow rates caused by pressure differentials in the reservoir, which are caused by factors other than the injection of injection and push waters and which hinder the performances. The specifications of the tracers, usable in these injection and push waters for optimizing oil recovery comprise the following characteristics:
Regarding the state of the art relating to such tracers for injection and push waters (tracing fluid) making it possible to probe oil reservoirs by diffusion between an injection well and a production well, one can cite the U.S. Pat. Nos. 4,231,426-B1 and 4,299,709-B1, which disclose aqueous tracer fluids comprising from 0.01 to 10% in weight of a nitrate salt associated with a bactericidal agent chosen among aromatic compounds (benzene, toluene, xylene). The Canadian patent application CA 2 674 127-A1 relates to a method consisting in using a natural isotope of carbon 13 for identifying premature drilling of injection waters in oil wells. In addition, there are a dozen families of molecules adapted and currently identified as tracers for injection waters in oil reservoirs. These molecule families are, for example, fluorinated benzoic acids or naphtalenesulfonic acids. The tracer molecules which are known and used have a specific chemical/radioactive signature. These known tracers can be detected with great sensitivity. However, they have three major drawbacks:
Furthermore, the site “ However, to date, no study has shown the feasibility of the detection of particles for oil tracing. It seems to be even generally accepted that particles, due to the volume of space they fill, are filtered and strongly retained in the soil, thus preventing any reasonable use of their detection as tracing. It is actually based on this hypothesis that the above-mentioned PowerPoint presentation from IFE is based. In fact, this document does not make any reference to the detection of particles, but rather to the detection of a by-product or a residue related to the degradation of these particles. In a completely different field, the French patent application FR 2 867 180-A1 describes hybrid nanoparticles comprising, on the one hand, a core made of rare earth oxides, optionally doped with rare earth or actinide or a mixture of rare earths or a mixture of rare earths and actinide, and, on the other hand, a coating around this core, said coating chiefly consisting of polysiloxane functionalized by at least one biological ligand grafted by covalent bonding. The core can be made of Gd2O3 doped with an amount of Tb3+ or by uranium and the polysiloxane coating can be achieved by making an aminopropyltriethoxysilane, a tetraethylsilicate, and a triethylamine react. These nanoparticles are used as probes for the detection, the monitoring, and the quantification of biological systems. The French patent application FR 2 922 106-A1 relates to the same technical field and targets the use of these nanoparticles as radiosensitizing agents for making the radiotherapy more efficient. The size of these nanoparticles is comprised between 10 and 50 nanometers. In this context, the present invention aims at meeting at least one of the following objectives:
These objectives, among others, are achieved by the invention which firstly relates to a method of study of a solid medium, for example an oil reservoir, by diffusion of a liquid through said solid medium, characterized in that it essentially consists in:
The invention secondly relates to an injection liquid in an oil reservoir usable particularly in the above-mentioned method characterized in that it comprises a nanoparticle-based tracer:
The invention thirdly relates to the use of nanoparticles such as defined hereinabove, as tracers in injection water of an oil reservoir, which are intended for the study of said reservoir by diffusion through the latter, in view particularly of monitoring the flows between an injection well and a production well and/or of evaluating the volumes of oil in reserve in the reservoir. This new technology according to the invention:
Method of Study of a Solid Medium The solid medium is preferably an oil reservoir contained in a subsoil (e.g. rocks) which can be of varied geological nature. The Nanoparticles: The size analysis of the nanoparticles of the tracer is, for example, measured by means of a commercial granulometer, namely: a Malvern granulometer, the Zetasizer Nano-S, based on PCS (Photon Correlation Spectroscopy). These nanoparticles are detectable, which means that their presence, or lack thereof, can be identified in the medium beyond a certain concentration and that their concentration can even be quantified as long as they are present in the medium. These nanoparticles are adapted to form a stable colloidal suspension in a saline medium, which does not settle much. For example, this suspension does not present any precipitation or agglomeration as time goes by, i.e., after 6 months at room temperature. According to an advantageous embodiment of this method, the core of the nanoparticles contains:
this matrix including within itself and/or at its surface:
Interactions To monitor the interactions between, on the one hand, the solid medium to be studied, namely, for example, the geological sub-soil (i.e. rocks) containing the oil reservoir and, on the other hand, the nanoparticles, it is possible, according to an advantageous provision of the invention, to adjust the HLB and/or the Zeta potential of the nanoparticle coating comprising the tracer based on said nanoparticles as a function of the solid medium to be studied. To do so, for example, either a same surface charge for the nanoparticles and the rocks of the solid medium is provided in order to create a repulsion and limit interactions, or the respective charges are modulated so the nanoparticles and the rocks of the solid medium interact in a controlled and/or specific manner with respect to certain rocks. Methodology According to a remarkable embodiment of the method according to the invention, prior to the analysis of the liquid having diffused, the latter is concentrated, preferably by filtration or dialysis, and, even more preferably, by tangential filtration and preferably by the use of a membrane using cutoffs of less than 100 kDa. To measure the quantity of tracer in the liquid having diffused, detection by fluorescence and/or by chemical analysis and/or by ICP and/or by magnetic analysis (magnetic transition temperature, for example of Curie or Néel) is favored. According to a variation for measuring the quantity of tracer in the liquid having diffused, one proceeds with at least one detection by time-resolved fluorescence, that is, triggered with delay after excitation (i.e. a few microseconds), which eliminates a large portion of the luminescence, intrinsic to the studied solid medium and measures only that relative to the tracing nanoparticle. Signals S to be Detected Preferably, each of at least one portion of the tracing nanoparticles comprises at least two (preferably two) signals to be detected:
Injection Liquid (Waters) for the Study of a Solid Medium, i.e. an Oil Reservoir According to another one of these objects, the invention relates to a new injection liquid in an oil reservoir usable, in particular, in the method defined hereinabove, characterized in that it comprises a nanoparticle-based tracer:
Advantageously, this liquid comprises water and the nanoparticles which it contains are such as defined hereinabove. Use of the Nanoparticles According to another one of these objects, the invention relates to a new use of the nanoparticles such as defined hereinabove as tracers in injection waters of an oil reservoir intended for the study of said reservoir by diffusion of these injection waters through said reservoir, particularly for monitoring the flows between an injection well and a production well and/or evaluating the volumes of oil in reserve in the reservoir. In a 2.5 ml bottle, 20 mg of FITC (fluorescein-isothiocyanate) and of APTES ((3-AminoPropyl) TriEthoxySilane) are inserted and vigorously agitated. After 30 minutes of homogenization at room temperature, the synthesis by microemulsion can begin. In a 100 ml Erlenmeyer flask, 2.27 ml of Triton X-100 (surfactant), 2.31 ml of n-hexanol (co-surfactant), 9.61 ml of cyclohexane and 0.61 ml of water with a pH of 5.5 are inserted and vigorously agitated. After 5 minutes, 0.040 ml of solution containing the fluorescein is added to the microemulsion, then 0.020 ml of APTES and 0.289 ml of TEOS (TetraEthOxyXilane). The silica polymerization reaction is completed by the addition of 0.173 ml of NH4OH (aqueous solution at 25%) after 30 minutes. The microemulsion system continues during 24 hours under agitation at room temperature. After 24 hours, the particles formed ( In a 2.5 ml bottle, 20 mg of fluorescein (FITC) and of APTES are inserted and vigorously agitated. After 30 minutes of homogenization at room temperature, the synthesis by microemulsion can begin. In a 100 ml Erlenmeyer flask, 2.10 ml of Triton X-100 (surfactant), 2.14 ml of n-hexanol (co-surfactant), 8.91 ml of cyclohexane (oil), and 1.4 ml of aqueous solution containing 0.595 ml of 16.7 mM HAuCl4.3H2O, 0.595 ml of 32.8 mM MES (sodium-2-mercaptoethanesulfonate) and 0.198 ml of 412 mM NaBH4, are inserted and vigorously agitated. After 5 minutes, 0.040 ml of solution containing the fluorescein is added to the microemulsion, then 0.020 ml of APTES and 0.289 ml of TEOS (TetraEthOxyXilane). The silica polymerization reaction is completed by the addition of 0.173 ml of NH4OH after 30 minutes. The microemulsion system continues during 24 hours while agitated at room temperature. After 24 hours, the particles formed ( In a 10 ml bottle, 400 mg of DTPA (DiethyleneTriaminePentaAcetic acid), 360 mg of EuCl37H2O and 5 ml of water whose pH is adjusted to be 8, are inserted. In a 100 ml Erlenmeyer flask, 2.27 ml of Triton X-100 (surfactant), 2.31 ml of n-hexanol (co-surfactant), 9.61 ml of cyclohexane, and 0.60 ml of the solution containing the DTPA and Eu3+ are inserted and vigorously agitated. After 5 minutes, 0.200 ml of TEOS is added to the microemulsion. The silica polymerization reaction is completed by the addition of 0.050 ml of a solution of NH4OH after 30 minutes. The microemulsion system is maintained during 24 hours under agitation at room temperature. After 24 hours, the particles formed are recovered by precipitation in centrifugation tubes by adding the same volume of ethanol for each wash. The washes are repeated 3 times with absolute ethanol. The obtained powder is finally dispersed in 20 ml of distilled water. In a 10 ml bottle, 500 mg of 2,6-pyridine dicarboxylic acid, 173 mg of N-HydroxySuccinimide (NHS), 287 mg of N-(3-dimethylaminopropyl)-N′-EthylCarboDiimide (EDC) hydrochloride are inserted in 5 ml of water whose pH is adjusted to be 8 (solution A). After 1 hour, 350 ml of APTES is added to the solution which is vigorously agitated for 90 minutes. In a 100 ml Erlenmeyer flask, 2.27 ml of Triton X-100 (surfactant), 2.31 ml of n-hexanol (co-surfactant), 9.61 ml of cyclohexane, and 0.60 ml of the solution A are inserted and vigorously agitated. After 5 minutes, 0.060 ml of APTES and 0.200 ml of TEOS are added to the microemulsion. The silica polymerization reaction is completed by the addition of 0.050 ml of NH4OH after 30 minutes. The microemulsion system is maintained during 24 hours under agitation at room temperature. After 24 hours, the particles formed are recovered by precipitation in centrifugation tubes by adding the same volume of ethanol for each wash. The washes are repeated 3 times with absolute ethanol. The obtained powder is finally dispersed in 20 ml of distilled water, then 360 mg of TbCl3 is added. In a 2.5 ml bottle, 20 mg of FITC and of APTES are inserted and vigorously agitated. After 30 minutes of homogenization at room temperature, the synthesis by microemulsion can begin. In a 100 ml Erlenmeyer flask, 2.27 ml of Triton X-100 (surfactant), 2.31 ml of n-hexanol (co-surfactant), 9.61 ml of cyclohexane and 0.61 ml of water with a pH of 5.5 are inserted and vigorously agitated. After 5 minutes, 0.040 ml of solution containing the fluorescein is added to the microemulsion, then 0.020 ml of APTES and 0.289 ml of TEOS. The silica polymerization reaction is completed by the addition of 0.173 ml of NH4OH after 30 minutes. The microemulsion system is maintained for 24 hours under agitation at room temperature. After 24 hours, 0.100 ml of TEOS and 0.050 ml of THPMP (3-TriHydroxysilylPropylMethylPhosphonate of sodium) are added to the microemulsion. After 24 hours under agitation, the particles formed are recovered by precipitation in centrifugation tubes by adding the same volume of ethanol for each wash. The washes are repeated three times with absolute ethanol. The obtained powder is finally dispersed in 20 ml of distilled water. In a 2.5 ml bottle, 20 mg of FITC and of APTES are inserted and vigorously agitated. After 30 minutes of homogenization at room temperature, the synthesis by microemulsion can begin. In a 100 ml Erlenmeyer flask, 2.27 ml of Triton X-100 (surfactant), 2.31 ml of n-hexanol (co-surfactant), 9.61 ml of cyclohexane (oil) and 0.61 ml of water with a pH of 5.5 are inserted and vigorously agitated. After 5 minutes, 0.040 ml of solution containing the fluorescein is added to the microemulsion, then 0.020 ml of APTES and 0.289 ml of TEOS (TetraEthOxyXilane). The silica polymerization reaction is completed by the addition of 0.173 ml of a solution of NH4OH after 30 minutes. The microemulsion system is maintained for 24 hours under agitation at room temperature. After 24 hours, 0.100 ml of TEOS and 40 mg of mPEG-Si (methoxy-polyethylene-glycol-triethoxysilane) are added to the microemulsion. After 24 hours under agitation, the particles formed are recovered by precipitation in centrifugation tubes by adding the same volume of ethanol for each wash. The washes are repeated three times with absolute ethanol. The obtained powder is finally dispersed in 20 ml of distilled water. In a 2.5 ml bottle, 20 mg of FITC and of APTES are inserted and vigorously agitated. After 30 minutes of homogenization at room temperature, the synthesis by microemulsion can begin. In a 100 ml Erlenmeyer flask, 2.27 ml of Triton X-100 (surfactant), 2.31 ml of n-hexanol (co-surfactant), 9.61 ml of cyclohexane and 0.61 ml of water with a pH of 5.5 are inserted and vigorously agitated. After 5 minutes, 0.040 ml of solution containing the fluorescein is added to the microemulsion, then 0.020 ml of APTES and 0.289 ml of TEOS. The silica polymerization reaction is completed by the addition of 0.173 ml of a solution of NH4OH after 30 minutes. The microemulsion system is maintained for 24 hours under agitation at room temperature. After 24 hours, 0.100 ml of TEOS and 0.100 ml of TMPTA (N-TriMethoxysilylPropyl-N,N,N-TrimethylAmmonium chloride) are added to the microemulsion. After 24 hours under agitation, the particles formed are recovered by precipitation in centrifugation tubes by adding the same volume of ethanol for each wash. The washes are repeated three times with absolute ethanol. The obtained powder is finally dispersed in 20 ml of distilled water. The preparation 8 consists in fabricating a cartridge allowing for a fluid to diffuse through a cylindrical core of porous rock in the longitudinal direction, without fluid loss on the side of the latter. The equipment used is composed of the core, two plugs having the same diameter, machined specifically for threaded, of a tube made of transparent polyvinyl chloride (PVC), of a pattern made of PolyTetraFluoroEthylene (PTFE), of Araldite® glue, and of a tube of commercial silicon joint. Push down one of the two plugs in the pattern, fix it with silicone, and then let dry for 30 minutes. Prepare the Araldite® glue in a small aluminum cup, then place the core on the plug and glue it, let dry for a few minutes. Do the same with the top plug. Cut the PVC tube to the corresponding length, put some silicone on the base of the tube, then turn it over on the pattern. Place everything in an incubator at 50° C. for ½ hour. Determine the volume of epoxy resin taking into account the phenomenon of imbibition in the rock (volume equivalent to 0.4 cm of diameter of the column) The epoxy resin is composed at 70% of a resin base (Epon® 828—Miller-Stephenson Chemical Company, Inc) and at 30% of a hardener (Versamid® 125—Miller-Stephenson Chemical Company, Inc). In a tumbler, mix the resin with the hardener for 10 minutes, then place the mixture at 50° C. for 40 to 50 minutes until a transparent and fluid mixture has been obtained. Slowly poor the mixture along the PVC tube, then leave at room temperature for two hours. Then, place the assembly at 70° C. for two hours. The preparation 9 is a diluted suspension of nanoparticles in sea water, which is the fluid used for the tests of diffusion through a porous core. A synthetic sea water solution is composed of demineralized water in which the following salts have been dissolved: Concentrated suspensions of nanoparticles according to the examples of preparation 1 to 7, are implemented. A certain quantity of these suspensions is diluted in a volume of sea water of 500 mL so as to end up with a final concentration in particles comprised between 5 and 20 mg/L. To this suspension, 0.5 g of potassium iodide is added, the potassium iodide having a 1 tracer behavior that is idea for the diffusion tests. The final suspension is then vacuum-degassed for 5 to 10 minutes. The assembly allowing for testing the diffusion of nanoparticles through a porous rock core and the functioning of this assembly are described here. A diluted suspension of particles according to preparation 1. A cartridge containing the porous rock is prepared according to preparation 8. The assembly is composed of a dual syringe pump allowing for fixing a flow rate comprised between 1 and 1000 mL per hour. The latter pumps the suspension of particles and directs it toward the cartridge containing the porous rock. The fluid diffuses through the latter, the differential pressure on both sides of the rock is monitored by a sensor. The fluid is finally directed toward a fraction collector. What is measured with these fractions is, on the one hand, the UV absorption at λ=254 nm of the fluid. The latter is very low when the fluid contains no iodide and becomes more important when the latter is present. The UV absorption at: =254 nm thus makes it possible to follow the diffusion of the ideal tracer. On the other hand, in the case of nanoparticles prepared according to the examples of preparation 1 or 2 or 5 or 6 or 7, the fluorescence is measured at λ=515 nm (excitation at 475 nm). The latter thus makes it possible to follow the diffusion of the particles. If several experiments are carried out on the same core, a step of counter-current washing by passage of a quantity greater than 500 mL of sea water obtained according to preparation 2 and without tracer, is provided. This example is a test of diffusion through a porous core cartridge according to preparation 8. The porous core is of the DU30804 type and has the following characteristics:
The suspension of nanoparticles has been obtained according to preparation 9. The flow rate imposed by the pump is 20 mL/hour. The fractions recovered at the exit of the rock have a volume of 5 mL. This example is a test of diffusion through a porous core cartridge according to preparation 8. The porous core is of the DU30804 type and has the following characteristics:
The suspension of nanoparticles has been obtained according to preparation 9. The flow rate imposed by the pump is 20 mL/hour. The fractions recovered at the exit of the rock have a volume of 5 mL. This example is a test of diffusion through a porous core cartridge according to preparation 8. The porous core is of the DU30804 type and has the following characteristics:
The suspension of nanoparticles has been obtained according to preparation 9. The flow rate imposed by the pump is 20 mL/hour. The fractions recovered at the exit of the rock have a volume of 5 mL. This example is a test of diffusion through a porous core cartridge according to preparation 8. The porous core is of the DU30804 type and has the following characteristics:
The suspension of nanoparticles has been obtained according to preparation 9. The flow rate imposed by the pump is 20 mL/hour. The fractions recovered at the exit of the rock have a volume of 5 mL. Comments: the curves 3 to 6 show the comparative curves of diffusion between the reference tracer (yellow) and the nanoparticles obtained according to the preparations 1, 5, 6, and 7, respectively. It is clearly shown that the particles corresponding to The present invention relates to the development of tracer fluids, more generally, that of aqueous liquids, intended to be injected under pressure in an oil reservoir, for example from an injection well up to a production well. The object of the invention is to propose a new method of study of a solid medium, i.e. an oil reservoir, by diffusion of a liquid (i.e. injection waters) containing tracers, through said solid medium, which is simple to implement and economical and which remedies the drawbacks of the known tracers for injection waters of oil reservoirs. This method essentially consisting of injecting, in this solid medium, an injection liquid comprising a nanoparticle-based tracer having average dimensions comprised between 20 and 200 nm, detectable by means of one or several S signals at dilutions of less than or equal to 10−7, adapted to form a stable colloidal suspension in a saline medium, at least a portion of which is constituted of a core and a coating provided with an adjustable hydrophilic-lipophilic balance (HLB) and comprising at least one organic and/or organosilicon component; recovering the liquid having diffused; and analyzing this liquid having diffused to measure the quantity of tracer by detection of the signal or signals S. 1. A method of study of a solid medium (i.e. an oil reservoir) by diffusion of a fluid through said solid medium, comprising:
injecting, in this solid medium (diffusion), a liquid (injection liquid) comprising a nanoparticle-based tracer;
having average dimensions comprised, in preferred ascending order, between 20 and 200 nm, 20 and 100 nm, 50 and 100 nm, 60 and 80 nm; detectable by means of one or several S signals at dilutions of less than or equal to 10; adapted to form a stable colloidal suspension in a saline medium; at least a portion of which is constituted of a core and a coating provided with an adjustable hydrophilic-lipophilic balance (HLB) and comprising at least one organic and/or organosilicon component. recovering the liquid having diffused; analyzing this liquid having diffused to measure the quantity of tracer by detection of the signal or signals S. 2. A method according to at least one material selected from the group consisting of: the semiconductors, noble metals, fluorides, vanadates or rare earth oxides and their mixtures and/or alloys; or a matrix selected from the group of materials consisting of: silicas, polysiloxanes, aluminas, zirconias, aluminates, aluminophosphates, metal oxides and their mixtures and/or alloys. this matrix including within itself and/or at its surface:
i. luminescent entities selected from the group consisting of: the semiconductors, oxides, rare earth fluorides or vanadates, organic fluorescent molecules, transition metal ions, rare earth ions connected, or not, to complexing molecules and/or to molecules allowing for enhancing their absorption and their mixtures and/or alloys; ii. optionally, other entities allowing for modifying the luminescence properties and selected from the group consisting of: noble metal particles and their mixtures and/or alloys; iii. and mixtures of (i) and (ii). 3. A method according to v. optionally charged hydrophilic compounds; vi. neutral hydrophilic compounds; vii. a hydrophobic polymer; viii. or of mixtures thereof. 4. A method according to 5. A method according to 6. A method according to 7. A method according to 8. A method according to 9. A method according to a signal S1 that can be emitted by fluorescent compounds and/or by chemiluminescent compounds and measured by fluorescence and/or by chemilimunescence; a signal S2 that can be emitted by a noble metal (such as gold, silver, platinum, and their mixtures and/or alloys), and measured by chemical analysis and/or ICP and/or magnetic analysis and/or susceptibility measurement, and/or measures of transition temperatures, and/or by analysis of FC/ZFC curves; said noble metal preferably constituting the core of the nanoparticle. 10. An injection liquid in an oil reservoir, usable in a method according to having dimensions comprised between 20 and 200 nm; detectable by means of one or several S signals at dilutions of less than or equal to 10−7; adapted to form a stable colloidal suspension in a saline medium; at least a portion of which is constituted of a core and a coating provided with an adjustable hydrophilic-lipophilic balance (HLB) and/or an adjustable Zeta potential and comprising at least one organic and/or organosilicon component. the hydrophilic-lipophilic balance (HLB) and/or a Zeta potential of said liquid which can also be adjustable. 11. A liquid according to 12. A method of monitoring flow between an injection well and a production well comprising injecting the nanoparticles defined in 13. A method of monitoring flow between an injection well and a production well comprising injecting the nanoparticles defined in TECHNOLOGICAL BACKGROUND
TECHNICAL PROBLEM AND OBJECTIVES TO ACHIEVE
BRIEF DESCRIPTION OF THE INVENTION
the hydrophilic-lipophilic balance (HLB) and/or a Zeta potential of said liquid which can also be adjustable.
DETAILED DESCRIPTION OF THE INVENTION
Regarding the coating of the nanoparticles, it is preferable for it to comprise grafted R-radicals, preferably by covalence, preferably based on surface silanes Si—R connections and originating from:
Advantageously, this nanoparticle coating comprises grafted R-radicals at the rate of at least one R-radical for 10 nm2 of surface, preferably at least one for 1 nm2.
the hydrophilic-lipophilic balance (HLB) and/or a Zeta potential of said liquid which can also be adjustable.
EXAMPLES
DESCRIPTION OF THE DRAWINGS
PREPARATION 1. SILICA PARTICLES (DIAMETER 64 NM) ENCAPSULATING FLUORSCEIN MOLECULES
PREPARATION 2. SILICA PARTICLES (DIAMETER 58 NM) OF THE CORE-SHELL TYPE WITH A GOLD CORE (DIAMETER 6 NM) AND A SILICA SHELL ENCAPSULATING FLUORSCEIN MOLECULES
PREPARATION 3. SILICA PARTICLES (DIAMETER 64 NM) ENCAPSULATE POLYDENTATE CHELATES OF EU3+
PREPARATION 4. SILICA PARTICLES (DIAMETER 64 NM) ENCAPSULATING CHELATES OF THE TERBIUM PYRIDINE TYPE
PREPARATION 5. GRAFTING OF PHOSPHONATE FUNCTIONS ON PARTICLES OF SILICA (DIAMETER 64 NM) ENCAPSULATING FLUORESCEIN MOLECULES
PREPARATION 6. GRAFTING OF UNCHARGED POLYETHYLENE-GLYCOL FUNCTIONS ON PARTICLES OF SILICA (DIAMETER 64 NM) ENCAPSULATING FLUORESCEIN MOLECULES
PREPARATION 7. GRAFTING OF AMINE FUNCTIONS ON PARTICLES OF SILICA (DIAMETER 64 NM) ENCAPSULATING FLUORESCEIN MOLECULES
PREPARATION 8. PREPARATION OF A SOLID MEDIUM CONSTITUTED BY A CARTRIDGE OF POROUS CORE
PREPARATION 9. PREPARATION OF A SUSPENSION OF NANOPARTICLES
NaCl 24.80 KCl 0.79 MgCl2 5.25 CaCl2 1.19 NaHCO3 0.20 Na2SO4 4.16 PREPARATION 10. ASSEMBLY FOR THE DIFFUSION OF A SUSPENSION OF NANOPARTICLES THROUGH A SOLID MEDIUM FORMED BY A CORE ACCORDING TO PREPARATION 8—OPERATION OF THIS ASSEMBLY
Example 1
Particles Prepared According to the Example of Preparation 1; Naked SiO2 Surface
Example 2
Particles Prepared According to the Example of Preparation 5; SiO2 Surface Grafted with Propyl-Methylphosphonates Groups
Example 3
Particles Prepared According to the Example of Preparation 6; SiO2 Surface Grafted with Polyethylene-Glycol, O-Methyl Groups
Example 4
Particles Prepared According to the Example of Preparation 7; SiO2 Surface Grafted with Propylamine Groups
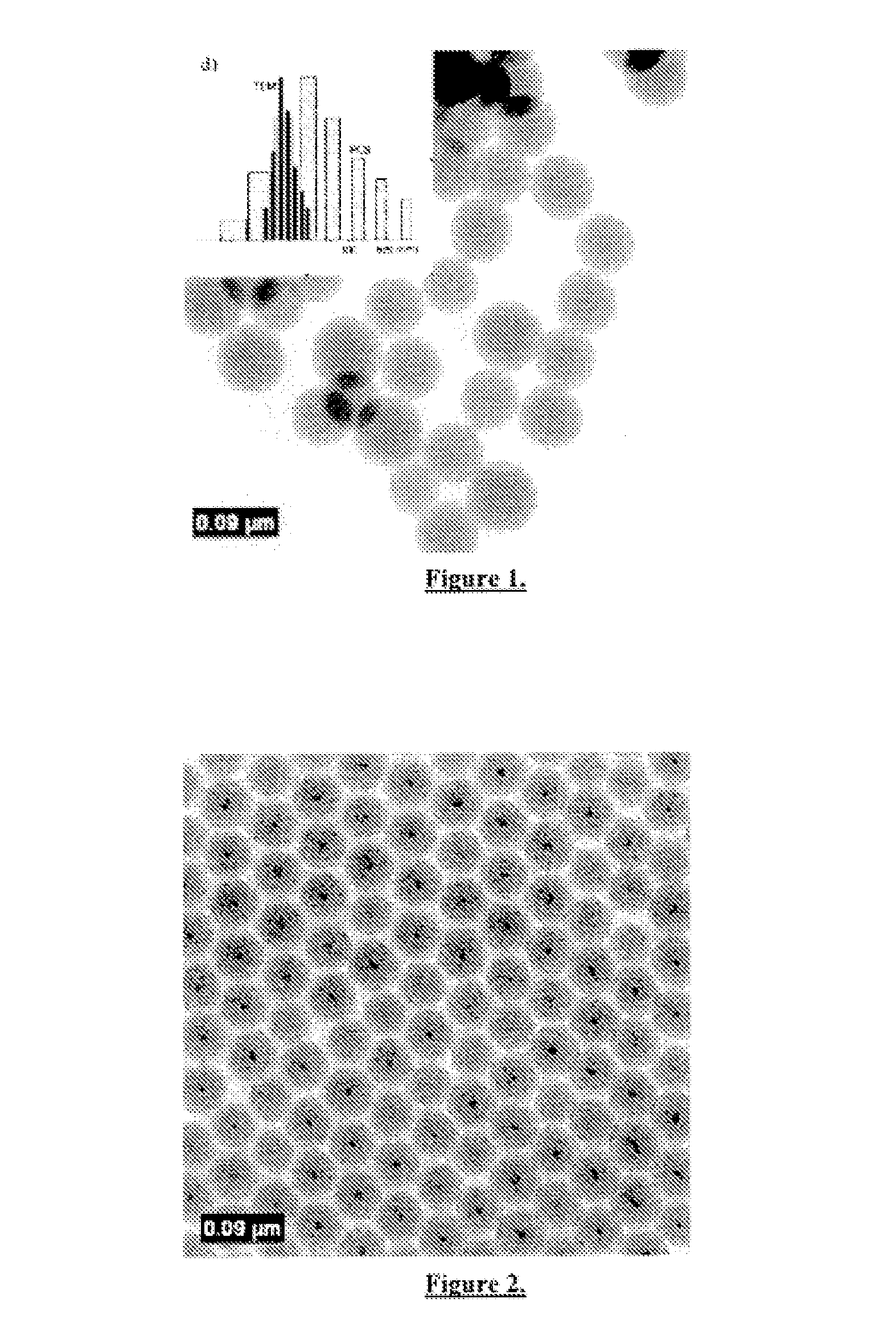
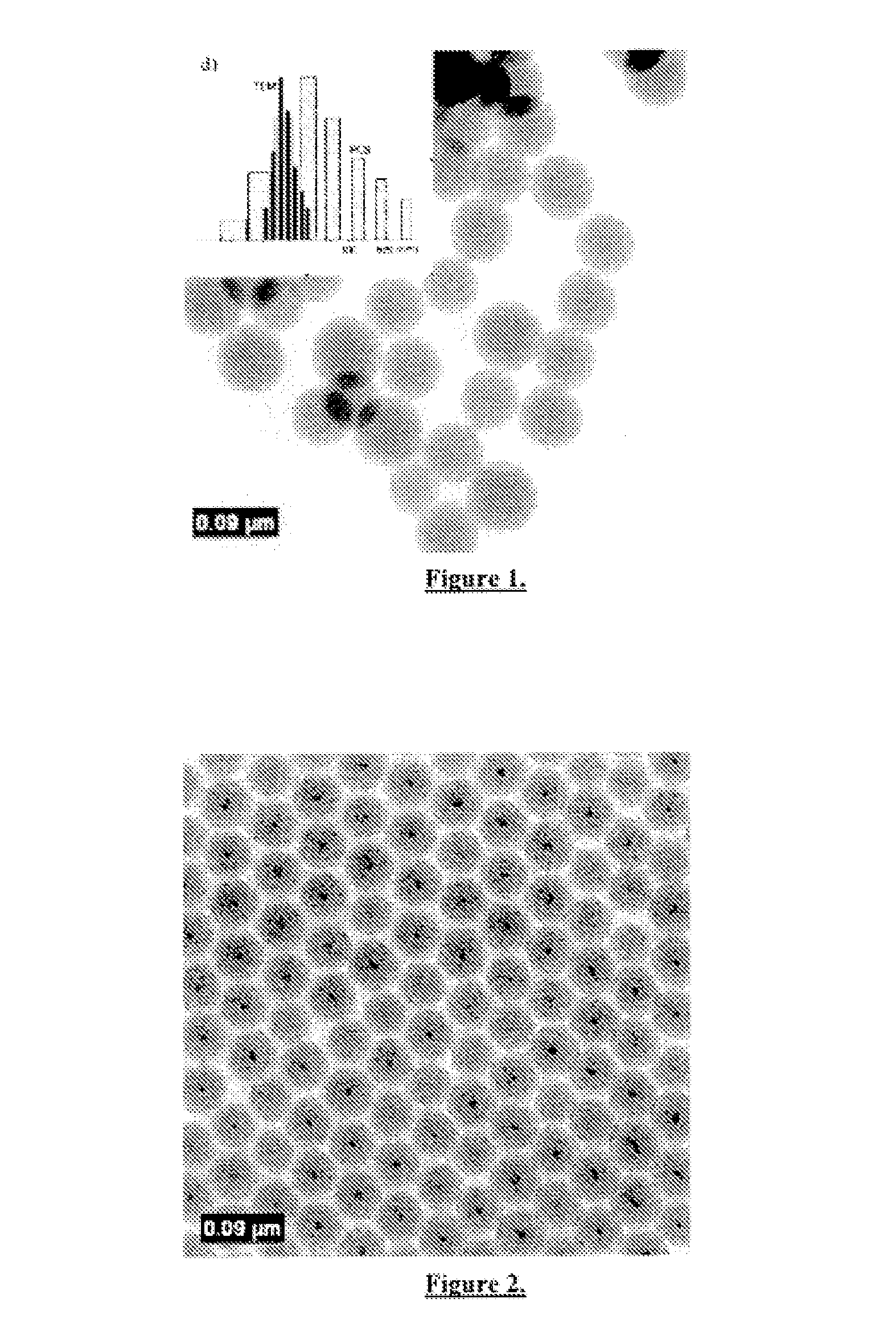
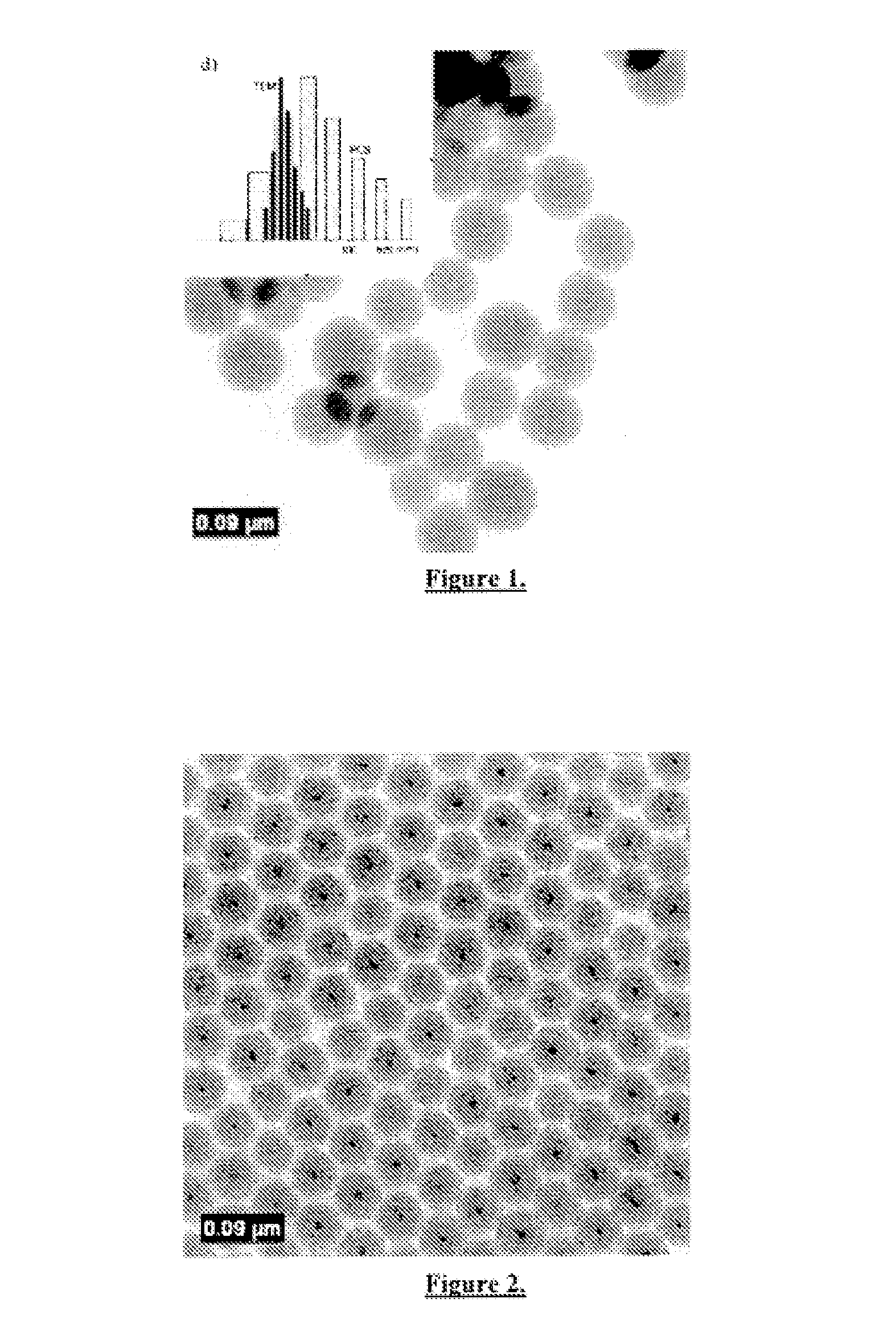